Home / 3 Factors That Stabilize Free Radicals
Free Radical Reactions
3 Factors That Stabilize Free Radicals
Last updated: July 6th, 2025 |
What Factors Affect Free-Radical Stability?
In the last article we introduced free radicals – neutral, electron-deficient chemical species with a partially filled orbital – and learned that they are highly reactive intermediates in organic chemistry. (See article – Free Radical Reactions)
In this post we’ll cover two of the most important concepts concerning these species: their geometry, and their stability. It’s this latter concept that we’ll see is particularly important for understanding many free-radical reactions in organic chemistry. [Spoiler: the factors that affect free radical stability are largely the same factors that stabilize carbocations [discussed previously here]
Table of Contents
- The Stability of Free Radicals Increases In The Order Methyl < Primary < Secondary < Tertiary
- Free Radicals Are Stabilized By Delocalization (“Resonance”)
- The Geometry Of Free Radicals Is That Of A “Shallow Pyramid” Which Allows For Overlap Of The Half-Filled p-Orbital With Adjacent Pi Bonds
- The Same Factors Which Stabilize Free Radicals Also Stabilize Carbocations
- Notes
- Quiz Yourself!
- (Advanced) References and Further Reading
1. Stability Of Free Radicals Increases In The Order Methyl < Primary < Secondary < Tertiary
Let’s talk a bit about stability first, and then circle back to their structure. Being electron deficient, you might already have a hunch regarding factors that might stabilize free radicals. Waaaay back, we talked about how a considerable portion of organic chemistry can be explained simply by understanding that: 1) opposite charges attract (and like charges repel), and 2) the stability of charges increases if it can be spread out over a greater volume. These still apply here!
Electron poor species are stabilized by neighboring atoms that can donate electron density. [“if you’re poor, it helps to have rich neighbors”].
The most common way to interpret “rich neighbors” here is the observation that increasing the number of alkyl groups on the carbon bearing the free radical increases its stability.
Radical stability increases in the order methyl < primary < secondary < tertiary. [For a second, more conceptually complex example, see the bottom of the post]. [Note 1]
2. Free Radicals Are Stabilized by Delocalization (“Resonance”)
Secondly, we have also learned that any factor which can lead to the electron deficient site being delocalized [spread out] over a larger area will also stabilize electron poor species. Previously, for example, we’ve seen that the positive charge of a carbocation is considerably stabilized when it is adjacent to a π bond.
That’s because the carbocation is sp2 hybridized and bears an empty p orbital, allowing for overlap with the adjacent p orbitals and therefore leading the positive charge to be delocalized over multiple carbon atoms, in a manner that is most easily grasped by drawing resonance structures.
Carbocations are flat – so it’s easy to see how the p orbital could be in line with adjacent p orbitals of a double bond. But what about the geometry of free radicals?
3. The Geometry of Free Radicals Is That Of A “Shallow Pyramid”, Which Allows For Overlap Of The Half-Filled p-Orbital With Adjacent Pi Bonds
If we draw out the electrons in a typical alkyl free radical, we see that there are three bonding pairs and a single unpaired electron, for a total of four occupied orbitals. By analogy to, say, amines, we might expect that the hybridization of the molecule to be sp3 and geometry of a free radical would be trigonal pyramidal.
In fact, the geometry of simple alkyl radicals is very close to flat and is often described as a “shallow pyramid”, with only a slight deviation (~ 5°) from planarity. [Note 2]
When the free radical is adjacent to a π bond, there’s a significant stabilization to be obtained if the p orbitals are all in line so they can overlap [i.e. be “in conjugation”] with each other. Overlap is increased (and the molecule’s energy lowered) if the “shallow pyramid” is flattened out.
It’s a good approximation to think of a free radical adjacent to a π bond as being sp2 -hybridized.
So what does this all boil down to? The electron-deficient free radical can be delocalized over multiple carbons. Therefore, free radicals are stabilized by resonance.
4. The Same Factors Which Stabilize Free Radicals Also Stabilize Carbocations
If you read the article on the stabilization of carbocations (See article – 3 Factors That Stabilize Carbocations) you might notice something: the same factors which stabilize free radicals are also the same factors which stabilize carbocations!
Quiz time: one of the most stable free radicals known is the triphenylmethyl radical, discovered by Moses Gomberg in 1900. In the absence of oxygen, this radical is indefinitely stable at room temperature. Can you identify the factors which might make this free radical particularly stable?
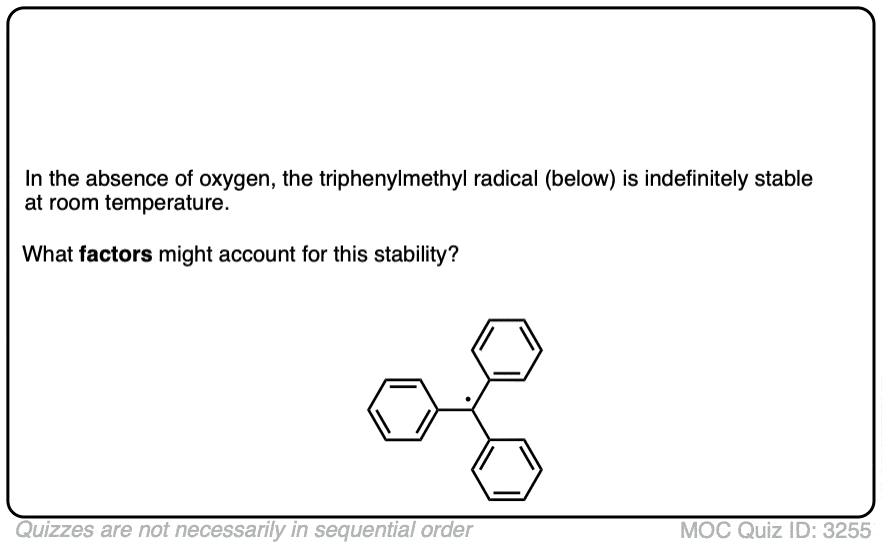
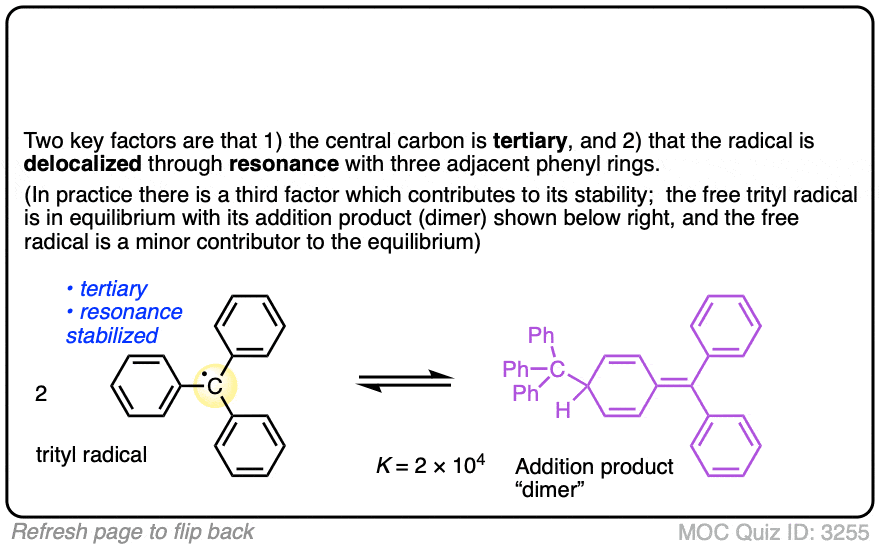
5. Removing Electron Density Destabilizes Free Radicals:
If we remember that free radicals are stabilized by electron donating groups, we might reason that they are destabilized when electron density is taken away.
So what factors might result in a free radical being less “electron rich” ?
There are three major factors. I’ll list them in order of importance for the purposes of a typical student encountering free radicals in a typical class.
6. Radical Stability Decreases With Increasing s-Character Of The Orbital
If you recall some of the factors that affect acidity you might recall that a lone pair of electrons becomes more stable as the hybridization of the carbon goes from sp3 to sp2 to sp. That’s because of the greater s-character of the orbital, which results in the lone pair being held more closely to the (positively charged) nucleus.
What might happen if we’re dealing with a free radical instead? As the s-character of the orbital containing the free radical is increased, the half-filled orbital containing the free radical is held more closely to the nucleus. What effect does this have on the stability of the free radical? It’s actually destabilizing, because being closer to the nucleus, the electron affinity of the orbital will increase.
For that reason alkyl radicals (generally considered to be sp2 hybridized) are the most stable, followed by vinyl and phenyl radicals (sp-hybridized) , followed by alkynyl radicals.
7. Radical Stability Decreases With Increasing Electronegativity of The Atom
Quiz time: Having read the paragraph above, what might you think is the effect of electronegativity on free radical stability? What might happen to the stability of a free radical as you increase the electronegativity of the atom? For example, compare the sequence H3C , H2N, HO, and F . Which free radical should be the most stable?
Electronegativity, as we’ve talked about before, is like “greed” for electrons. Increasing electronegativity is going to draw a free radical closer to the nucleus, and as we saw above, this results in destabilization.
8. Radical Stability Decreases As Polarizability Is Decreased
Going down the periodic table, we also notice an increasing stability in free radicals, going from F < Cl < Br < I . While this can likewise be thought of as resulting from a decrease in electronegativity , another way to look at it is that going down the periodic table results in an increase in the size of the atom, and with that, allows for the electron-deficient orbital to be spread out over a greater volume.
9. Summary: Factors Which Stabilize (and Destabilize) Free Radicals
The main factor we’ve seen here that destabilizes free radicals is bringing the half-filled orbital closer to the nucleus (greater s-character, higher electronegativity) or by restricting the delocalization of the free radical (decreasing polarizability). Here, I think it’s important not to focus on the effect of electronegativity on the electron (radical) but on the effect of electronegativity on the “hole” – i.e. the empty orbital. Bringing a half-empty orbital closer to the nucleus will greatly increase its potential energy (the electrostatic attraction of the nucleus for an electron) and increase electron affinity, making that free radical much more reactive (and in this case, reactivity = instability).
So how might we “quantify” the stability of a free radical?
Believe it or not, there’s actually a remarkably simple way to learn how stable free radicals are, using a measurement you’re probably already familiar with! We’ll talk about that in the next post.
Next Post: Bond Strengths And Radical Stability
Notes
Related Articles
- Bond Strengths And Radical Stability
- 3 Factors That Stabilize Carbocations
- Free Radical Initiation: Why Is “Light” Or “Heat” Required?
- Initiation, Propagation, Termination
- Monochlorination Products Of Propane, Pentane, And Other Alkanes
- In Summary: Free Radicals
- Free Radicals Practice Quizzes (MOC Membership required)
Note 1. Stabilization of Free Radicals By Adjacent Lone Pairs
In addition to alkyl groups, free radicals are also stabilized by adjacent groups with lone pairs, such as oxygen and nitrogen. At first thought, oxygen might not seem like much of an electron donating group, since it’s quite electronegative. However, oxygen does have two lone pairs of electrons. How might these be involved?
The adjacent oxygen atom can donate electron density to the half-empty p orbital, which is a stabilizing interaction. The orbital picture looks like this.
Yes, there’s an electron in the antibonding orbital, but on the whole the interaction is stabilizing since bonding electrons outnumber antibonding electrons here.
Note 2. One note for advanced students – the “shallow pyramid” has a low barrier to inversion (1-2 kcal/mol). This means that if a free radical is formed from an optically active chiral center, rapid racemization generally ensues.
From Carey & Sundberg A, 4th ed. p. 676:
“Simple alkyl radicals are generally pyramidal, although the barrier to inversion is very small…. theoretical results also indicate that the barrier to inversion is no more than 1-2 kcal/mol, so rapid inversion will occur”.
From the same book (p. 675):
“The IR spectrum of the methyl radical has been recorded at very low temperatures in frozen argon. This IR study puts a maximum of ~5° on the deviation from planarity…”
Note 3. A previous version of this post included adjacent electron-withdrawing groups as a destabilizing influence on free-radical stability. I’ve removed this because it’s just too complicated for our purposes.
For example, take the methyl radical, H3C• . Replacing hydrogen with fluorine (a strong electron withdrawing group) one might expect radical stability to decrease. Well, it doesn’t. It’s actually more stable due to the ability of the fluorine lone pair to donate to the half-filled orbital. A second fluorine has a similar effect. However, the F3C• radical is less stable than the methyl radical. This is hard to predict with simple rules. See: Homolytic Bond Dissociation Enthalpies of the C−H Bonds Adjacent to Radical Centers, Xian-Man Zhang The Journal of Organic Chemistry 1998 63 (6), 1872-1877 . DOI: 10.1021/jo971768d
A note on hybridization. Vinyl radicals have an (E) and (Z) form and the inversion barrier from one to the other increases as the electronegativity of the substituents increases. Since the molecule must pass through an sp-hybridized geometry in order to invert, this supports the notion that taking electron density away from the sp-orbital destabilizes the radical. This is a very interesting paper where the rates of inversion were studied:
Effect of Substituents on the Structure of the Vinyl Radical: Calculations and Experiments. Carlo Galli,*, Alessandra Guarnieri,Heinz Koch,†, Paolo Mencarelli,* and, and Zvi Rappoport‡ The Journal of Organic Chemistry 1997 62 (12), 4072-4077 DOI: 10.1021/jo962373h
Quiz Yourself!
Become a MOC member to see the clickable quiz with answers on the back.
Become a MOC member to see the clickable quiz with answers on the back.
Become a MOC member to see the clickable quiz with answers on the back.
Become a MOC member to see the clickable quiz with answers on the back.
Become a MOC member to see the clickable quiz with answers on the back.
Become a MOC member to see the clickable quiz with answers on the back.
Become a MOC member to see the clickable quiz with answers on the back.
(Advanced) References and Further Reading
A useful table of C-H and C-C bond dissociation energies (a useful proxy for radical stabilities) can be found here:
- AN INSTANCE OF TRIVALENT CARBON: TRIPHENYLMETHYL.
Moses Gomberg
Journal of the American Chemical Society 1900, 22 (11), 757-771
DOI: 10.1021/ja02049a006
This is regarded as the famous paper that started the field of organic free-radical chemistry. Moses Gomberg (University of Michigan) was attempting to synthesize hexaphenylethane, and so tried reducing triphenylmethyl chloride (trityl chloride) with Zn metal. Instead, he got a yellow solution which gave a product incorporating oxygen when exposed to air. When he conducted this experiment under a CO2 blanket (remember, pure Ar was not readily available back then!), he found that the solution was indefinitely stable, and deduced that it was the triphenylmethyl radical, which reacted with oxygen when exposed to air, forming a peroxide. At the end of the paper, Gomberg states “This work will be continued and I wish to reserve the field for myself”. The ACS declared the University of Michigan as a national historical chemical landmark in 2000, celebrating the centenary of this discovery, and Prof. Melanie Sanford (U Michigan) currently holds the Moses Gomberg chair in Chemistry. - Theoretical Interpretation of Carbon‐13 Hyperfine Interactions in Electron Spin Resonance Spectra
Martin Karplus and George K. Fraenkel
J. Chem. Phys. 1961, 35, 1312
DOI: 10.1063/1.1732044
The EPR (electron paramagnetic resonance) spectrum of the methyl radical leads to the conclusion that its structure could be either planar or a very shallow pyramid. Prof. Karplus is now at Harvard and received the Nobel Prize in Chemistry in 2013 for his contributions to theoretical chemistry. - Structure of the tert-butyl radical
L. Bonazzola, N. Leray, and J. Roncin
Journal of the American Chemical Society 1977, 99 (25), 8348-8349
DOI: 10.1021/ja00467a052 - Configuration of the tert-butyl radical
D. Griller, K. U. Ingold, P. J. Krusic, and H. Fischer
Journal of the American Chemical Society 1978, 100 (21), 6750-6752
DOI: 10.1021/ja00489a035
The t-butyl radical has been studied extensively. Whereas experimental results have been interpreted in terms of both planar and slightly pyramidal structures, theoretical calculations favor a pyramidal structure. - Reactions of Atoms and Free Radicals in Solution. I. A Study of the Substitution of Hydrogen on an Asymmetric Carbon Atom—The Chlorination of Primary Active Amyl Chloride
Herbert C. Brown, M. S. Kharasch, and T. H. Chao
Journal of the American Chemical Society 1940, 62 (12), 3435-3439
DOI: 1021/ja01869a040 - N-Bromosuccinimide. III. Stereochemical Course of Benzylic Bromination
H. J. Dauben and Layton L. McCoy
Journal of the American Chemical Society 1959, 81 (20), 5404-5409
DOI: 10.1021/ja01529a038
The above two papers demonstrate that radical reactions at chiral carbon centers leads to racemization, because of the easy inversion of the intermediate pyramidal radical. - Ab Initio Calculations of the Relative Resonance Stabilization Energies of Allyl and Benzyl Radicals
David A. Hrovat and Weston Thatcher Borden
The Journal of Physical Chemistry 1994, 98 (41), 10460-10464
DOI: 1021/j100092a014
The stabilization energy of a vinyl group (in the allyl radical) and a phenyl group (in the benzyl radical) has been calculated to be 15.7 kcal/mol and 12.5 kcal/mol, respectively. - Effects of adjacent acceptors and donors on the stabilities of carbon-centered radicals
G. Bordwell, Xianman Zhang, and Mikhail S. Alnajjar
Journal of the American Chemical Society 1992, 114 (20), 7623-7629
DOI: 10.1021/ja00046a003
Table I in this paper contains stabilization energies of methyl radicals with various substituents (e.g. ·CH2X). - Infrared Spectrum of the Methyl Radical in Solid Argon
L. Andrews and G.C. Pimentel,
J. Chem. Phys. 1967, 47, 3637
DOI: 10.1063/1.1712434
This study puts a maximum of about 5° on the deviation of the methyl radical from planarity.
00 General Chemistry Review
01 Bonding, Structure, and Resonance
- How Do We Know Methane (CH4) Is Tetrahedral?
- Hybrid Orbitals and Hybridization
- How To Determine Hybridization: A Shortcut
- Orbital Hybridization And Bond Strengths
- Sigma bonds come in six varieties: Pi bonds come in one
- A Key Skill: How to Calculate Formal Charge
- The Four Intermolecular Forces and How They Affect Boiling Points
- 3 Trends That Affect Boiling Points
- How To Use Electronegativity To Determine Electron Density (and why NOT to trust formal charge)
- Introduction to Resonance
- How To Use Curved Arrows To Interchange Resonance Forms
- Evaluating Resonance Forms (1) - The Rule of Least Charges
- How To Find The Best Resonance Structure By Applying Electronegativity
- Evaluating Resonance Structures With Negative Charges
- Evaluating Resonance Structures With Positive Charge
- Exploring Resonance: Pi-Donation
- Exploring Resonance: Pi-acceptors
- In Summary: Evaluating Resonance Structures
- Drawing Resonance Structures: 3 Common Mistakes To Avoid
- How to apply electronegativity and resonance to understand reactivity
- Bond Hybridization Practice
- Structure and Bonding Practice Quizzes
- Resonance Structures Practice
02 Acid Base Reactions
- Introduction to Acid-Base Reactions
- Acid Base Reactions In Organic Chemistry
- The Stronger The Acid, The Weaker The Conjugate Base
- Walkthrough of Acid-Base Reactions (3) - Acidity Trends
- Five Key Factors That Influence Acidity
- Acid-Base Reactions: Introducing Ka and pKa
- How to Use a pKa Table
- The pKa Table Is Your Friend
- A Handy Rule of Thumb for Acid-Base Reactions
- Acid Base Reactions Are Fast
- pKa Values Span 60 Orders Of Magnitude
- How Protonation and Deprotonation Affect Reactivity
- Acid Base Practice Problems
03 Alkanes and Nomenclature
- Meet the (Most Important) Functional Groups
- Condensed Formulas: Deciphering What the Brackets Mean
- Hidden Hydrogens, Hidden Lone Pairs, Hidden Counterions
- Don't Be Futyl, Learn The Butyls
- Primary, Secondary, Tertiary, Quaternary In Organic Chemistry
- Branching, and Its Affect On Melting and Boiling Points
- The Many, Many Ways of Drawing Butane
- Wedge And Dash Convention For Tetrahedral Carbon
- Common Mistakes in Organic Chemistry: Pentavalent Carbon
- Table of Functional Group Priorities for Nomenclature
- Summary Sheet - Alkane Nomenclature
- Organic Chemistry IUPAC Nomenclature Demystified With A Simple Puzzle Piece Approach
- Boiling Point Quizzes
- Organic Chemistry Nomenclature Quizzes
04 Conformations and Cycloalkanes
- Staggered vs Eclipsed Conformations of Ethane
- Conformational Isomers of Propane
- Newman Projection of Butane (and Gauche Conformation)
- Introduction to Cycloalkanes
- Geometric Isomers In Small Rings: Cis And Trans Cycloalkanes
- Calculation of Ring Strain In Cycloalkanes
- Cycloalkanes - Ring Strain In Cyclopropane And Cyclobutane
- Cyclohexane Conformations
- Cyclohexane Chair Conformation: An Aerial Tour
- How To Draw The Cyclohexane Chair Conformation
- The Cyclohexane Chair Flip
- The Cyclohexane Chair Flip - Energy Diagram
- Substituted Cyclohexanes - Axial vs Equatorial
- Ranking The Bulkiness Of Substituents On Cyclohexanes: "A-Values"
- Cyclohexane Chair Conformation Stability: Which One Is Lower Energy?
- Fused Rings - Cis-Decalin and Trans-Decalin
- Naming Bicyclic Compounds - Fused, Bridged, and Spiro
- Bredt's Rule (And Summary of Cycloalkanes)
- Newman Projection Practice
- Cycloalkanes Practice Problems
05 A Primer On Organic Reactions
- The Most Important Question To Ask When Learning a New Reaction
- Curved Arrows (for reactions)
- Nucleophiles and Electrophiles
- The Three Classes of Nucleophiles
- Nucleophilicity vs. Basicity
- What Makes A Good Nucleophile?
- What Makes A Good Leaving Group?
- 3 Factors That Stabilize Carbocations
- Equilibrium and Energy Relationships
- 7 Factors that stabilize negative charge in organic chemistry
- 7 Factors That Stabilize Positive Charge in Organic Chemistry
- What's a Transition State?
- Hammond's Postulate
- Learning Organic Chemistry Reactions: A Checklist (PDF)
- Introduction to Oxidative Cleavage Reactions
06 Free Radical Reactions
- Free Radical Reactions
- 3 Factors That Stabilize Free Radicals
- Bond Strengths And Radical Stability
- Free Radical Initiation: Why Is "Light" Or "Heat" Required?
- Initiation, Propagation, Termination
- Monochlorination Products Of Propane, Pentane, And Other Alkanes
- Selectivity In Free Radical Reactions
- Selectivity in Free Radical Reactions: Bromination vs. Chlorination
- Halogenation At Tiffany's
- Allylic Bromination
- Bonus Topic: Allylic Rearrangements
- In Summary: Free Radicals
- Synthesis (2) - Reactions of Alkanes
- Free Radicals Practice Quizzes
07 Stereochemistry and Chirality
- Types of Isomers: Constitutional Isomers, Stereoisomers, Enantiomers, and Diastereomers
- How To Draw The Enantiomer Of A Chiral Molecule
- How To Draw A Bond Rotation
- Introduction to Assigning (R) and (S): The Cahn-Ingold-Prelog Rules
- Assigning Cahn-Ingold-Prelog (CIP) Priorities (2) - The Method of Dots
- Enantiomers vs Diastereomers vs The Same? Two Methods For Solving Problems
- Assigning R/S To Newman Projections (And Converting Newman To Line Diagrams)
- How To Determine R and S Configurations On A Fischer Projection
- The Meso Trap
- Optical Rotation, Optical Activity, and Specific Rotation
- Optical Purity and Enantiomeric Excess
- What's a Racemic Mixture?
- Chiral Allenes And Chiral Axes
- Stereochemistry Practice Problems and Quizzes
08 Substitution Reactions
- Nucleophilic Substitution Reactions - Introduction
- Two Types of Nucleophilic Substitution Reactions
- The SN2 Mechanism
- Why the SN2 Reaction Is Powerful
- The SN1 Mechanism
- The Conjugate Acid Is A Better Leaving Group
- Comparing the SN1 and SN2 Reactions
- Polar Protic? Polar Aprotic? Nonpolar? All About Solvents
- Steric Hindrance is Like a Fat Goalie
- Common Blind Spot: Intramolecular Reactions
- Substitution Practice - SN1
- Substitution Practice - SN2
09 Elimination Reactions
- Elimination Reactions (1): Introduction And The Key Pattern
- Elimination Reactions (2): The Zaitsev Rule
- Elimination Reactions Are Favored By Heat
- Two Elimination Reaction Patterns
- The E1 Reaction
- The E2 Mechanism
- E1 vs E2: Comparing the E1 and E2 Reactions
- Antiperiplanar Relationships: The E2 Reaction and Cyclohexane Rings
- Bulky Bases in Elimination Reactions
- Comparing the E1 vs SN1 Reactions
- Elimination (E1) Reactions With Rearrangements
- E1cB - Elimination (Unimolecular) Conjugate Base
- Elimination (E1) Practice Problems And Solutions
- Elimination (E2) Practice Problems and Solutions
10 Rearrangements
11 SN1/SN2/E1/E2 Decision
- Identifying Where Substitution and Elimination Reactions Happen
- Deciding SN1/SN2/E1/E2 (1) - The Substrate
- Deciding SN1/SN2/E1/E2 (2) - The Nucleophile/Base
- SN1 vs E1 and SN2 vs E2 : The Temperature
- Deciding SN1/SN2/E1/E2 - The Solvent
- Wrapup: The Key Factors For Determining SN1/SN2/E1/E2
- Alkyl Halide Reaction Map And Summary
- SN1 SN2 E1 E2 Practice Problems
12 Alkene Reactions
- E and Z Notation For Alkenes (+ Cis/Trans)
- Alkene Stability
- Alkene Addition Reactions: "Regioselectivity" and "Stereoselectivity" (Syn/Anti)
- Stereoselective and Stereospecific Reactions
- Hydrohalogenation of Alkenes and Markovnikov's Rule
- Hydration of Alkenes With Aqueous Acid
- Rearrangements in Alkene Addition Reactions
- Halogenation of Alkenes and Halohydrin Formation
- Oxymercuration Demercuration of Alkenes
- Hydroboration Oxidation of Alkenes
- m-CPBA (meta-chloroperoxybenzoic acid)
- OsO4 (Osmium Tetroxide) for Dihydroxylation of Alkenes
- Palladium on Carbon (Pd/C) for Catalytic Hydrogenation of Alkenes
- Cyclopropanation of Alkenes
- A Fourth Alkene Addition Pattern - Free Radical Addition
- Alkene Reactions: Ozonolysis
- Summary: Three Key Families Of Alkene Reaction Mechanisms
- Synthesis (4) - Alkene Reaction Map, Including Alkyl Halide Reactions
- Alkene Reactions Practice Problems
13 Alkyne Reactions
- Acetylides from Alkynes, And Substitution Reactions of Acetylides
- Partial Reduction of Alkynes With Lindlar's Catalyst
- Partial Reduction of Alkynes With Na/NH3 To Obtain Trans Alkenes
- Alkyne Hydroboration With "R2BH"
- Hydration and Oxymercuration of Alkynes
- Hydrohalogenation of Alkynes
- Alkyne Halogenation: Bromination and Chlorination of Alkynes
- Oxidation of Alkynes With O3 and KMnO4
- Alkenes To Alkynes Via Halogenation And Elimination Reactions
- Alkynes Are A Blank Canvas
- Synthesis (5) - Reactions of Alkynes
- Alkyne Reactions Practice Problems With Answers
14 Alcohols, Epoxides and Ethers
- Alcohols - Nomenclature and Properties
- Alcohols Can Act As Acids Or Bases (And Why It Matters)
- Alcohols - Acidity and Basicity
- The Williamson Ether Synthesis
- Ethers From Alkenes, Tertiary Alkyl Halides and Alkoxymercuration
- Alcohols To Ethers via Acid Catalysis
- Cleavage Of Ethers With Acid
- Epoxides - The Outlier Of The Ether Family
- Opening of Epoxides With Acid
- Epoxide Ring Opening With Base
- Making Alkyl Halides From Alcohols
- Tosylates And Mesylates
- PBr3 and SOCl2
- Elimination Reactions of Alcohols
- Elimination of Alcohols To Alkenes With POCl3
- Alcohol Oxidation: "Strong" and "Weak" Oxidants
- Demystifying The Mechanisms of Alcohol Oxidations
- Protecting Groups For Alcohols
- Thiols And Thioethers
- Calculating the oxidation state of a carbon
- Oxidation and Reduction in Organic Chemistry
- Oxidation Ladders
- SOCl2 Mechanism For Alcohols To Alkyl Halides: SN2 versus SNi
- Alcohol Reactions Roadmap (PDF)
- Alcohol Reaction Practice Problems
- Epoxide Reaction Quizzes
- Oxidation and Reduction Practice Quizzes
15 Organometallics
- What's An Organometallic?
- Formation of Grignard and Organolithium Reagents
- Organometallics Are Strong Bases
- Reactions of Grignard Reagents
- Protecting Groups In Grignard Reactions
- Synthesis Problems Involving Grignard Reagents
- Grignard Reactions And Synthesis (2)
- Organocuprates (Gilman Reagents): How They're Made
- Gilman Reagents (Organocuprates): What They're Used For
- The Heck, Suzuki, and Olefin Metathesis Reactions (And Why They Don't Belong In Most Introductory Organic Chemistry Courses)
- Reaction Map: Reactions of Organometallics
- Grignard Practice Problems
16 Spectroscopy
- Degrees of Unsaturation (or IHD, Index of Hydrogen Deficiency)
- Conjugation And Color (+ How Bleach Works)
- Introduction To UV-Vis Spectroscopy
- UV-Vis Spectroscopy: Absorbance of Carbonyls
- UV-Vis Spectroscopy: Practice Questions
- Bond Vibrations, Infrared Spectroscopy, and the "Ball and Spring" Model
- Infrared Spectroscopy: A Quick Primer On Interpreting Spectra
- IR Spectroscopy: 4 Practice Problems
- 1H NMR: How Many Signals?
- Homotopic, Enantiotopic, Diastereotopic
- Diastereotopic Protons in 1H NMR Spectroscopy: Examples
- 13-C NMR - How Many Signals
- Liquid Gold: Pheromones In Doe Urine
- Natural Product Isolation (1) - Extraction
- Natural Product Isolation (2) - Purification Techniques, An Overview
- Structure Determination Case Study: Deer Tarsal Gland Pheromone
17 Dienes and MO Theory
- What To Expect In Organic Chemistry 2
- Are these molecules conjugated?
- Conjugation And Resonance In Organic Chemistry
- Bonding And Antibonding Pi Orbitals
- Molecular Orbitals of The Allyl Cation, Allyl Radical, and Allyl Anion
- Pi Molecular Orbitals of Butadiene
- Reactions of Dienes: 1,2 and 1,4 Addition
- Thermodynamic and Kinetic Products
- More On 1,2 and 1,4 Additions To Dienes
- s-cis and s-trans
- The Diels-Alder Reaction
- Cyclic Dienes and Dienophiles in the Diels-Alder Reaction
- Stereochemistry of the Diels-Alder Reaction
- Exo vs Endo Products In The Diels Alder: How To Tell Them Apart
- HOMO and LUMO In the Diels Alder Reaction
- Why Are Endo vs Exo Products Favored in the Diels-Alder Reaction?
- Diels-Alder Reaction: Kinetic and Thermodynamic Control
- The Retro Diels-Alder Reaction
- The Intramolecular Diels Alder Reaction
- Regiochemistry In The Diels-Alder Reaction
- The Cope and Claisen Rearrangements
- Electrocyclic Reactions
- Electrocyclic Ring Opening And Closure (2) - Six (or Eight) Pi Electrons
- Diels Alder Practice Problems
- Molecular Orbital Theory Practice
18 Aromaticity
- Introduction To Aromaticity
- Rules For Aromaticity
- Huckel's Rule: What Does 4n+2 Mean?
- Aromatic, Non-Aromatic, or Antiaromatic? Some Practice Problems
- Antiaromatic Compounds and Antiaromaticity
- The Pi Molecular Orbitals of Benzene
- The Pi Molecular Orbitals of Cyclobutadiene
- Frost Circles
- Aromaticity Practice Quizzes
19 Reactions of Aromatic Molecules
- Electrophilic Aromatic Substitution: Introduction
- Activating and Deactivating Groups In Electrophilic Aromatic Substitution
- Electrophilic Aromatic Substitution - The Mechanism
- Ortho-, Para- and Meta- Directors in Electrophilic Aromatic Substitution
- Understanding Ortho, Para, and Meta Directors
- Why are halogens ortho- para- directors?
- Disubstituted Benzenes: The Strongest Electron-Donor "Wins"
- Electrophilic Aromatic Substitutions (1) - Halogenation of Benzene
- Electrophilic Aromatic Substitutions (2) - Nitration and Sulfonation
- EAS Reactions (3) - Friedel-Crafts Acylation and Friedel-Crafts Alkylation
- Intramolecular Friedel-Crafts Reactions
- Nucleophilic Aromatic Substitution (NAS)
- Nucleophilic Aromatic Substitution (2) - The Benzyne Mechanism
- Reactions on the "Benzylic" Carbon: Bromination And Oxidation
- The Wolff-Kishner, Clemmensen, And Other Carbonyl Reductions
- More Reactions on the Aromatic Sidechain: Reduction of Nitro Groups and the Baeyer Villiger
- Aromatic Synthesis (1) - "Order Of Operations"
- Synthesis of Benzene Derivatives (2) - Polarity Reversal
- Aromatic Synthesis (3) - Sulfonyl Blocking Groups
- Birch Reduction
- Synthesis (7): Reaction Map of Benzene and Related Aromatic Compounds
- Aromatic Reactions and Synthesis Practice
- Electrophilic Aromatic Substitution Practice Problems
20 Aldehydes and Ketones
- What's The Alpha Carbon In Carbonyl Compounds?
- Nucleophilic Addition To Carbonyls
- Aldehydes and Ketones: 14 Reactions With The Same Mechanism
- Sodium Borohydride (NaBH4) Reduction of Aldehydes and Ketones
- Grignard Reagents For Addition To Aldehydes and Ketones
- Wittig Reaction
- Hydrates, Hemiacetals, and Acetals
- Imines - Properties, Formation, Reactions, and Mechanisms
- All About Enamines
- Breaking Down Carbonyl Reaction Mechanisms: Reactions of Anionic Nucleophiles (Part 2)
- Aldehydes Ketones Reaction Practice
21 Carboxylic Acid Derivatives
- Nucleophilic Acyl Substitution (With Negatively Charged Nucleophiles)
- Addition-Elimination Mechanisms With Neutral Nucleophiles (Including Acid Catalysis)
- Basic Hydrolysis of Esters - Saponification
- Transesterification
- Proton Transfer
- Fischer Esterification - Carboxylic Acid to Ester Under Acidic Conditions
- Lithium Aluminum Hydride (LiAlH4) For Reduction of Carboxylic Acid Derivatives
- LiAlH[Ot-Bu]3 For The Reduction of Acid Halides To Aldehydes
- Di-isobutyl Aluminum Hydride (DIBAL) For The Partial Reduction of Esters and Nitriles
- Amide Hydrolysis
- Thionyl Chloride (SOCl2) And Conversion of Carboxylic Acids to Acid Halides
- Diazomethane (CH2N2)
- Carbonyl Chemistry: Learn Six Mechanisms For the Price Of One
- Making Music With Mechanisms (PADPED)
- Carboxylic Acid Derivatives Practice Questions
22 Enols and Enolates
- Keto-Enol Tautomerism
- Enolates - Formation, Stability, and Simple Reactions
- Kinetic Versus Thermodynamic Enolates
- Aldol Addition and Condensation Reactions
- Reactions of Enols - Acid-Catalyzed Aldol, Halogenation, and Mannich Reactions
- Claisen Condensation and Dieckmann Condensation
- Decarboxylation
- The Malonic Ester and Acetoacetic Ester Synthesis
- The Michael Addition Reaction and Conjugate Addition
- The Robinson Annulation
- Haloform Reaction
- The Hell–Volhard–Zelinsky Reaction
- Enols and Enolates Practice Quizzes
23 Amines
- The Amide Functional Group: Properties, Synthesis, and Nomenclature
- Basicity of Amines And pKaH
- 5 Key Basicity Trends of Amines
- The Mesomeric Effect And Aromatic Amines
- Nucleophilicity of Amines
- Alkylation of Amines (Sucks!)
- Reductive Amination
- The Gabriel Synthesis
- Some Reactions of Azides
- The Hofmann Elimination
- The Hofmann and Curtius Rearrangements
- The Cope Elimination
- Protecting Groups for Amines - Carbamates
- The Strecker Synthesis of Amino Acids
- Introduction to Peptide Synthesis
- Reactions of Diazonium Salts: Sandmeyer and Related Reactions
- Amine Practice Questions
24 Carbohydrates
- D and L Notation For Sugars
- Pyranoses and Furanoses: Ring-Chain Tautomerism In Sugars
- What is Mutarotation?
- Reducing Sugars
- The Big Damn Post Of Carbohydrate-Related Chemistry Definitions
- The Haworth Projection
- Converting a Fischer Projection To A Haworth (And Vice Versa)
- Reactions of Sugars: Glycosylation and Protection
- The Ruff Degradation and Kiliani-Fischer Synthesis
- Isoelectric Points of Amino Acids (and How To Calculate Them)
- Carbohydrates Practice
- Amino Acid Quizzes
25 Fun and Miscellaneous
- A Gallery of Some Interesting Molecules From Nature
- Screw Organic Chemistry, I'm Just Going To Write About Cats
- On Cats, Part 1: Conformations and Configurations
- On Cats, Part 2: Cat Line Diagrams
- On Cats, Part 4: Enantiocats
- On Cats, Part 6: Stereocenters
- Organic Chemistry Is Shit
- The Organic Chemistry Behind "The Pill"
- Maybe they should call them, "Formal Wins" ?
- Why Do Organic Chemists Use Kilocalories?
- The Principle of Least Effort
- Organic Chemistry GIFS - Resonance Forms
- Reproducibility In Organic Chemistry
- What Holds The Nucleus Together?
- How Reactions Are Like Music
- Organic Chemistry and the New MCAT
26 Organic Chemistry Tips and Tricks
- Common Mistakes: Formal Charges Can Mislead
- Partial Charges Give Clues About Electron Flow
- Draw The Ugly Version First
- Organic Chemistry Study Tips: Learn the Trends
- The 8 Types of Arrows In Organic Chemistry, Explained
- Top 10 Skills To Master Before An Organic Chemistry 2 Final
- Common Mistakes with Carbonyls: Carboxylic Acids... Are Acids!
- Planning Organic Synthesis With "Reaction Maps"
- Alkene Addition Pattern #1: The "Carbocation Pathway"
- Alkene Addition Pattern #2: The "Three-Membered Ring" Pathway
- Alkene Addition Pattern #3: The "Concerted" Pathway
- Number Your Carbons!
- The 4 Major Classes of Reactions in Org 1
- How (and why) electrons flow
- Grossman's Rule
- Three Exam Tips
- A 3-Step Method For Thinking Through Synthesis Problems
- Putting It Together
- Putting Diels-Alder Products in Perspective
- The Ups and Downs of Cyclohexanes
- The Most Annoying Exceptions in Org 1 (Part 1)
- The Most Annoying Exceptions in Org 1 (Part 2)
- The Marriage May Be Bad, But the Divorce Still Costs Money
- 9 Nomenclature Conventions To Know
- Nucleophile attacks Electrophile
27 Case Studies of Successful O-Chem Students
- Success Stories: How Corina Got The The "Hard" Professor - And Got An A+ Anyway
- How Helena Aced Organic Chemistry
- From a "Drop" To B+ in Org 2 – How A Hard Working Student Turned It Around
- How Serge Aced Organic Chemistry
- Success Stories: How Zach Aced Organic Chemistry 1
- Success Stories: How Kari Went From C– to B+
- How Esther Bounced Back From a "C" To Get A's In Organic Chemistry 1 And 2
- How Tyrell Got The Highest Grade In Her Organic Chemistry Course
- This Is Why Students Use Flashcards
- Success Stories: How Stu Aced Organic Chemistry
- How John Pulled Up His Organic Chemistry Exam Grades
- Success Stories: How Nathan Aced Organic Chemistry (Without It Taking Over His Life)
- How Chris Aced Org 1 and Org 2
- Interview: How Jay Got an A+ In Organic Chemistry
- How to Do Well in Organic Chemistry: One Student's Advice
- "America's Top TA" Shares His Secrets For Teaching O-Chem
- "Organic Chemistry Is Like..." - A Few Metaphors
- How To Do Well In Organic Chemistry: Advice From A Tutor
- Guest post: "I went from being afraid of tests to actually looking forward to them".
Hi, I have a question: Are charged species with an unpaired electron also free radicals?
There are such things as “radical anions”, which bear both a radical and a charge. One example is the ketyl radical anion that is formed from the reduction of benzophenone by sodium metal. https://en.wikipedia.org/wiki/Ketyl
Is there a free radical activity order for all kinds of radicals? For example,
……> Cl(.) > CH3(.) > Me3C(.) > …..
I don’t understand how do people make remarks on molecular orbitals of heteroatomic species in org. chem. Can somebody explain ?
Why is it that the order of stability of free radicals is allylic > benzylic > tertiary butyl, but is the exact opposite in case of carbocations?
how can factors stabilising radicals and carbocations be same?radicals can be stabilised by both electron donating and withdrawing groups right?
Hi James,
I have several questions regarding the reactivity of the allyl and benzyl radicals towards association with HO2: allyl/benzyl+ho2–>allyl-ho2/benzyl-ho2.
I have read several posts and papers claiming that although the benzyl radical shows more resonant structures than the allyl one, both radicals show similar stability because some of the resonant structures of benzyl are not very stable as they break the aromaticity. This makes me think that the reactivity of both radicals when it comes to associate with an HO2 radical is similar, and both reactions should show similar rate constants.
However, I wonder if this issue could be addressed in a different way. How about looking into the SOMO orbitals of allyl, benzyl, and HO2? I have run quantum calculations and observed that the energy of the SOMO of allyl is 21.9 kcal mol-1 with respect to that of the HO2 (set as 0.0 kcal mol-1 as a reference energy), while that of the benzyl radical is 28.9 kcal mol-1. The energy gap between the SOMO orbitals of the pair HO2-Allyl is 7.0 kcal mol-1 smaller than that of the HO2-Benzyl one. Does this mean that the overlap between the SOMO orbitals of allyl and HO2 is much larger and thus the resulting MO during the association reaction will be much more stable? If so, does this mean that the association reaction allyl+HO2 should show much larger rate constants than benzyl+HO2 reaction?
Thank you
I want to ask when O oxygen shows it +M group character and have free radical on itself by making double bond with tha carbon ,at that time numbers of electronic Oxygen is 9 which can not be possible with the 2nd period’s member….Please explain
Hi, I have questions about the MO diagram: why 4 MO orbitals? and why 6 electrons?
It’s simplified. The interaction is between a lone pair on oxygen and a half-filled orbital on carbon. There are only 3 electrons in the system. The middle section depicts the lowering of the energy of the lone pair, and the raising of the energy of the antibonding orbital. Overall the system is more stable.
Does steric hindrance have any affect in whether a radical will form? For example, would ethylbenzene be more likely to undergo radical halogenation than tert-butylbenzene?
Well, t-butylbenzene would certainly not form a radical as easily as ethylbenzene, but that’s because there are no hydrogens that can be removed that will result in a resonance-stabilized radical!
Generally speaking steric effects are not as important in free radical reactions.
One of the reasons is that charged nucleophiles and electrophiles carry around a solvent “shell” with them that helps to stabilize the charges. Kind of like an entourage that surrounds them at all times. Free radical reactions are neutral and this “entourage” effect is not as much of an issue.
hi..can i ask you one question..why is it when the benzylic undergoes monohalogenation..the halogen attach to the side chain insteaad of the ring itself?
please answer???
Look up the difference between aryl radicals and benzylic radicals. One is resonance stabilized, the other is not.
I want to ask which free radical is the most stable in following
a. Primary
b. Methyl
c. Secondary
d. Tertiary
Plz reply
Can free radicals undergo ring expansion
For our purposes, no.
In fact the cyclopropylmethyl radical is unusually stable, and although I don’t have the numbers at the moment the cyclobutylmethyl radical would also be more stable than an ordinary primary radical as well since it can interact with the “banana bonds” of the cyclobutane.
If I want to irradiate UV to polypropylene (PP, it has tertiary C) and polysulfone (PSf, it has quarternary C), would PSf form free-radical more easily?
Thanks for taking time to answer my question.
Yes PSf certainly would form a radical more easily since it has a chromophore that can be accessed with UV light. You’d get loss of SO2 and formation of two adjacent radicals which could recombine or go on to other chain reactions, depending on conditions.
Could you please explain why are there two unpaired electrons in the MO diagram at the end of your article? Shouldn’t it be only one?
Hi – this is an effect called “hyperconjugation”. It’s an interaction between a filled orbital (the lone pair of oxygen) and a half-empty orbital (the free radical). Donation of electron density to the half-filled orbital results in some stabilization. This is what the orbital picture is trying to present. I haven’t written an article on hyperconjgation yet but there is a wikipedia article.
Thanks for clarifying.
MO diagrams with unpaired electrons and molecules with more than two atoms are unfamiliar to me, so I’ll settle for that for now :)
This “hyperconjugation” that James was talking about is also the reason for the stability increase from primary to tertiary alkyl radicals; it’s worth noting that the lone pair on the oxygen and the two electrons in the sigma bond between the adjacent carbon and hydrogen are carrying out the same function, and aren’t separate entities.
I wouldn’t call it hyperconjugation since hyperconjugation is (by definition) between sigma bond and and empty or half empty orbital. A quantum phyical chemist would call it just conjugation. Even though it’s very unorthodox.
[Modern Physical Organic Chemistry By Eric Anslyn]