Home / m-CPBA (meta-chloroperoxybenzoic acid)
Alkene Reactions
m-CPBA (meta-chloroperoxybenzoic acid)
Last updated: February 16th, 2025 |
m-Chloroperoxybenzoic Acid (m-CPBA) For The Epoxidation of Alkenes
- m-CPBA (meta-chloroperoxybenzoic acid) is a useful reagent for the formation of epoxides from alkenes (note – often just called, m-chloroperbenzoic acid, without the “oxy”)
- In this reaction, the C-C pi bond is broken, and two new C-O single bonds are formed on the same face of the alkene pi-bond. Since both bonds are formed on the same face, this is an example of a syn addition.
- The weak O-O bond in m-CPBA is also broken. The -OH of the peroxyacid is the source of the oxygen in the new epoxide
- Epoxidation of alkenes with m-CPBA is an example of a stereospecific reaction. The configuration of atoms about the C–C bond is always conserved. The reaction proceeds through a concerted transition state.
- Other peroxyacids such as peracetic acid, perbenzoic acid, and trifluoroperacetic acid are also effective reagents for epoxidation of alkenes.
- Alkynes do not undergo reaction with m-CPBA to give epoxides
- m-CPBA is also useful for the Baeyer-Villiger oxidation, a reaction that converts ketones to esters [for more on this, see post – The Baeyer-Villiger Reaction]
Table of Contents
1. Epoxidation of Alkenes With Peroxyacids
When alkenes are treated with peroxyacids such as meta-chloroperoxybenzoic acid (m-CPBA), two new C-O bonds are formed and a C-C pi bond is broken, resulting in the formation of an epoxide. (An O–O bond from m-CPBA is also broken, which is the source of the oxygen in the epoxide. The byproduct is m-chlorobenzoic acid).
Epoxides, also known as oxiranes, are 3-membered cyclic ethers. Since the inner bond angles of epoxides (approximately 60°) deviate significantly from the preferred tetrahedral geometry around carbon (109.5°) , they possess considerable ring strain (about 13 kcal/mol) and undergo a large number of useful ring-opening reactions with nucleophiles. (For more on the reactions of epoxides, see Epoxides – the Outlier of the Ether Family]
Epoxidation of alkenes with peroxyacids such as m-CPBA always occurs in such a way that both C-O bonds are formed on the same face of the alkene, an outcome known as “syn addition”.
You can tell that addition is syn here because the new C-O bonds are both drawn as “wedges” (pointing out of the page) or “dashes” (pointing into the page).
In this case both of these products are identical, since rotating the molecule 180° results in the same product (see below)
via GIPHY (Look at how unhappy those plastic pieces are – the model kit really helps you visualize the ring strain :- ) )
In many other cases this will result in the formation of a pair of enantiomers or diasteromers, depending on the structure of the starting alkene.
Epoxidation of alkenes with peroxyacids never results in anti addition.
It is incorrect to draw the product of this epoxidation reaction as having one “wedge” C-O and one “dash” C-O, since this would represent anti addition.
(BTW if you try to make a model, you will see that this outcome would also be impossibly strained)
2. Epoxidation of Alkenes Is Stereospecific
In epoxidation reactions, the configuration of atoms about the alkene is always conserved.
For example, the trans alkene below gives only the trans product (as an equal mixture of enantiomers). Note that in product A, the trans arrangement of C–H bonds about the C–C bond has been conserved.
The product B where the two C-H bonds are cis to the C-C bond is not formed. (Note that this product B would be the diastereomer of product A).
Likewise, the cis alkene gives only product B. (Product A, diastereomers of product B, are not formed.)
Since the starting trans and cis alkenes differ only in their configuration but yield products which are stereoisomers, epoxidation of alkenes is therefore an example of a stereospecific reaction. [see IUPAC for the definition, also see article: Stereoselective and Stereospecific Reactions]
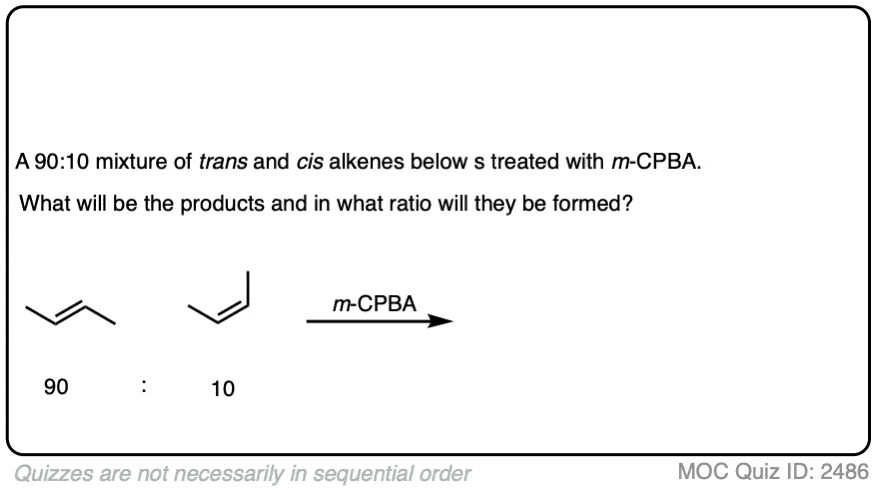
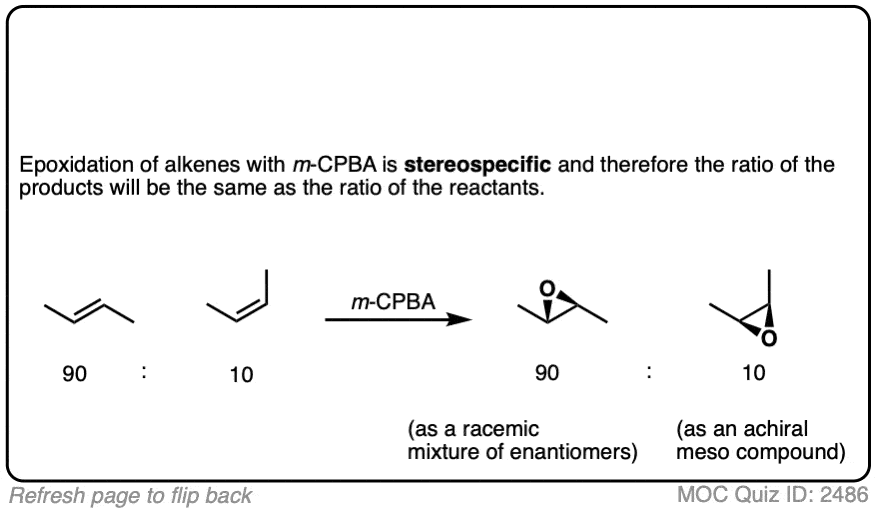
3. The Mechanism of Epoxidation of Alkenes With m-CPBA
Now that we’ve identified the bonds that form and break, and the expected stereochemistry for this reaction, we can start to ask ourselves how this reaction actually works.
First of all: which oxygen from m-CPBA is transferred to give the epoxide?
Isotopic labelling studies can verify that the oxygen of the epoxide comes from the OH of the peroxyacid, not the interior O connected to the C=O.
One driving force for the reaction is breakage of the relatively weak O–O and C-C pi bonds (bond dissocation energies of about 45 kcal/mol and 60 kcal/mol, respectively) in exchange for two relatively strong C-O sigma bonds (about 90 kcal/mol).
The transition state that has been proposed for the reaction of m-CPBA (and other peroxyacids) with alkenes has become known as the “butterfly mechanism”, owing to the four(!) partial bonds around the central oxygen that give the appearance of a large-winged insect.
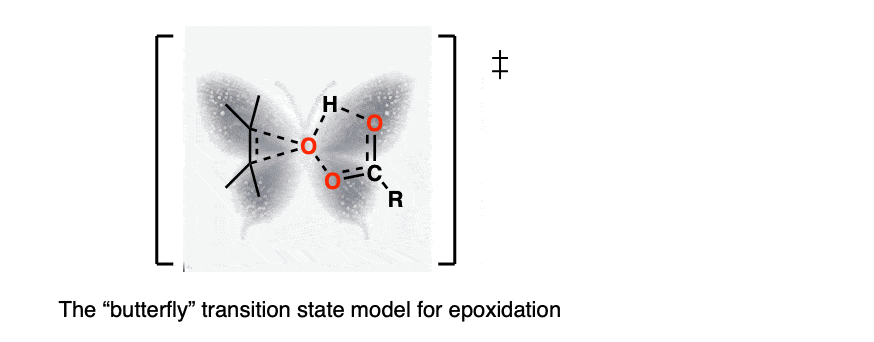
This reaction gets my vote for the busiest one-step arrow-pushing mechanism in all of introductory organic chemistry:
Let’s break down everything that happens here in this one (!) step:
- The C–C pi bond breaks
- Two new C–O single bonds form
- The (weak) O–OH bond breaks
- Meanwhile, the resulting carboxylic acid is transposed: a new C–O pi bond forms, while the existing C-O pi bond acts as a base to remove a proton from the terminal oxygen.
This “butterfly” mechanism accounts for the following experimental observations:
- The reaction is first-order in both peroxyacid and alkene (second order overall)
- The rate of reaction is not sensitive to the polarity of the solvent (making a charged carbocation intermediate unlikely) [Note 1]
It also accounts for the stereospecificity of the reaction. We would not expect a reaction that proceeds through a carbocation intermediate to be stereospecific, for example. (The reaction of HCl with alkenes is not stereospecific, for example)
Two additional facts are worth noting.
- Electron-rich alkenes react more quickly than electron poor alkenes [Ref], and
- adding electron-withdrawing groups to the R group of the peroxyacid make it more reactive. (The added chlorine on the 3-position of the benzene ring makes it more electron-withdrawing and therefore more reactive, relative to plain-ol’ peroxybenzoic acid).
4. Which Alkene Will React?
Let’s imagine we have a molecule containing multiple C-C pi bonds that is treated with m-CPBA.
Is it possible to get epoxidation to happen at just one alkene pi-bond, or do we just end up with a mixture of products?
What is observed is that the most electron-rich alkene undergoes epoxidation first, with remarkably good selectivity.
“Most electron-rich” generally means the alkene which has the most carbon atoms directly attached to the alkene (i.e. “most substituted”) so long as they are not electron-withdrawing groups.
For instance, in the alkene above, it’s the trisubstituted alkene preferentially undergoes epoxidation while the mono-substituted alkene is untouched.
This might seem counter-intuitive since we might expect that a more substituted alkene is more sterically hindered, but in practice the rate of reaction is more sensitive to electronic effects (i.e. electron-rich vs. electron-poor) than steric effects. [Note 2] [Ref]
See if you can apply this concept in the question below:
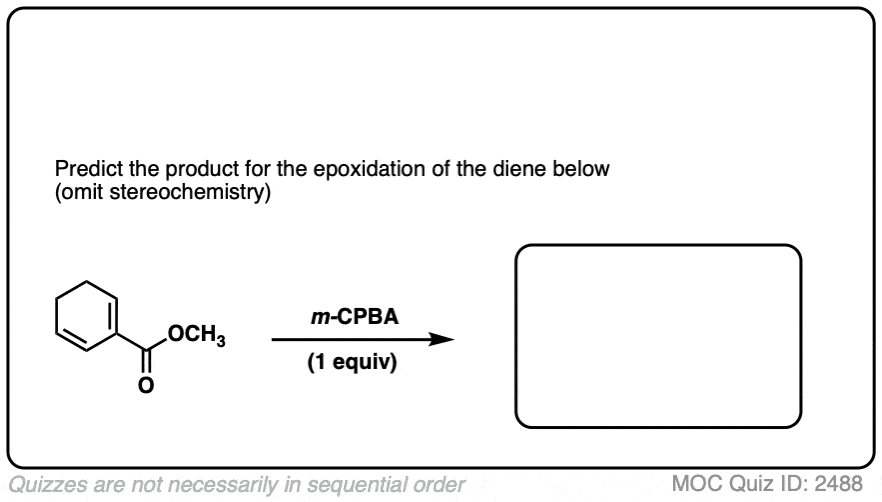
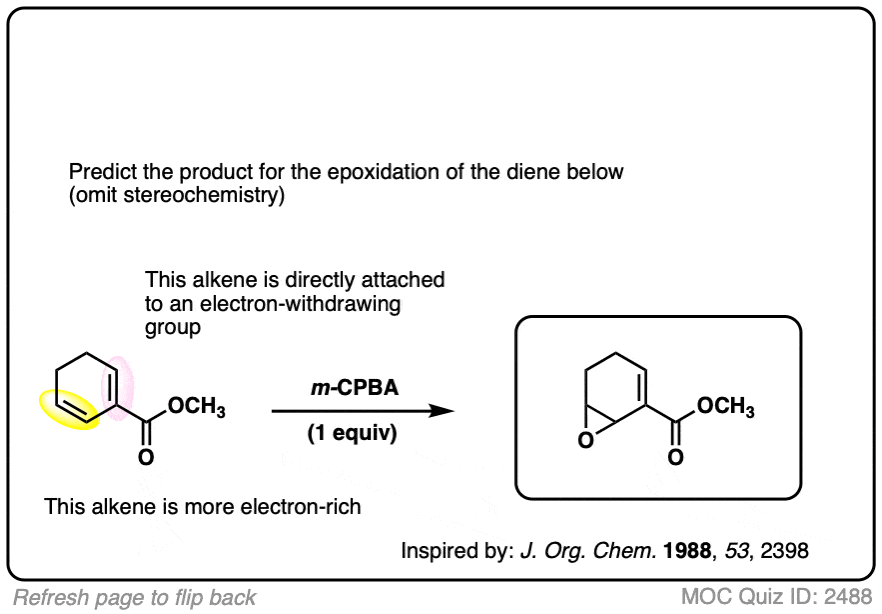
5. Stereoselectivity
When epoxidation is performed on an alkene containing pre-existing chiral centers, then there is the potential for the formation of stereoisomers. That’s because the two faces of the alkene will not be equivalent steric environments, as seen from the perspective of electrophile (such as m-CPBA).
A particularly striking example is the bicyclic alkene below. When it is treated with m-CPBA, a 99:1 mixture of products (diastereomers) is formed. The reason for the high selectivity is that the electrophile (m-CPBA) only encounters a single CH2 group in its approach to the top face (favored), whereas it encounters a two-carbon bridge in is approach on the bottom face (disfavored).
In this case, the result is a 99:1 ratio of products favoring attack on the least hindered face of the alkene.
There is a more systematic method for naming faces of alkenes, (known as Re and Si ) which we will not get into at present.
6. Other Epoxidation Reagents
Other peroxyacids have been used for epoxidation reactions, such as peroxyacetic acid, peroxybenzoic acid, and trifluoroperoxyacetic acid.
Just as making the alkene more electron-rich increases the rate of reaction, it has also been observed that adding electron-withdrawing reagents to the electrophile (peroxyacid) increases the rate of reaction.
The epoxidation of alkenes with peroxyacids has been known since 1909, but it was only in the 1960s that m-CPBA started gaining widespread use, which greatly improved the scope of epoxidation reactions. [Note 3]
Furthermore, although they aren’t often covered in introductory courses, there are other ways of epoxidizing alkenes that don’t involve peroxyacids. Examples include metals such as vanadium, titanium, manganese and others in the presence of peroxides (or hydroperoxides), or the use of reagents such as dimethyldioxirane (DMDO).
7. Sharpless Asymmetric Epoxidation
Enantioselective epoxidation of alkenes is also a known process.
In an enantioselective reaction, one starts with an achiral molecule and adds a chiral reagent that selectively attacks one face of the starting material over the other, resulting in a mixture of products which is enriched in on enantiomer over another. (One can’t just use any chiral reagent – there’s a lot of trial and error involved in getting the right recipe) [Note]
One prominent method for the enantioselective epoxidation of alkenes was developed by K. Barry Sharpless (Scripps) and has become known as the Sharpless epoxidation.
The Sharpless epoxidation involves treating an allylic alcohol with a witches’ brew of titanium isopropoxide [Ti(Oi-Pr)4)] t-butylhydroperoxide (the oxidant) and, depending on which enantiomer is desired, either (S,S) or (R, R) diethyl tartrate (or di-isopropyl tartrate, in some cases)
Here is a simple example.
The reaction is not enantioselective without the OH group on the carbon adjacent to the alkene. (This class of molecules is known as “allylic alcohols”). The hydroxyl group is required to coordinate to the titanium and direct the epoxidation.
For much more on the Sharpless epoxidation, I highly recommend these notes (Chemistry 115, Harvard University, Prof. Andrew G. Myers).
Notes
Related Articles
- Epoxides – The Outlier Of The Ether Family
- Epoxide Ring Opening With Base
- Opening of Epoxides With Acid
- OsO4 (Osmium Tetroxide) for Dihydroxylation of Alkenes
- Stereoselective and Stereospecific Reactions
- More Reactions on the Aromatic Sidechain: Reduction of Nitro Groups and the Baeyer Villiger
- What’s a Racemic Mixture?
- Alkene Addition Pattern #3: The “Concerted” Pathway
- Cycloalkanes – Ring Strain In Cyclopropane And Cyclobutane
- Palladium on Carbon (Pd/C) for Catalytic Hydrogenation
- Alkene Addition Reactions: “Regioselectivity” and “Stereoselectivity” (Syn/Anti)
For this article, Encyclopedia of Reagents for Organic Synthesis was very useful [See on archive.org] , as was March’s Advanced Organic Chemistry and Prof Andrew Myers’ Chem 115 Notes (Harvard).
Note 1. A typical solvent for epoxidation with m-CPBA is the relatively non-polar solvent dichloromethane. Interestingly, the rate of epoxidation is much slower in hydrogen-bonding solvents, as these tend to disrupt the internal hydrogen bonding of the “butterfly” transition state. [Ref]
Note 2. This reminds me of an old joke among organic chemistry graduate students: if you’re asked at a group meeting to explain why a particular reaction happens (or doesn’t happen), the stock answer is, “a combination of electronics and sterics”. Covers all the bases!
Note 3. The reactivity of m-CPBA is highly superior to that of peroxybenzoic acid, but only became widely available after 1963. For a comparison of the scope of peroxybenzoic acid and m-CPBA, compare [Ref] and [Ref] – m-CPBA gives cleaner and faster reactions, with greater scope. It is also a nicely crystalline white solid. Commercially available m-CPBA consists of about 80% m-CPBA with the remainder being m-chlorobenzoic acid. It can be purified further by treating with a buffer, but great care should be taken with highly purified m-CPBA due to its potentially explosive properties.
Note 4. To give an idea of the trial and error involved in the development of this reaction, see Sharpless’ Nobel Lecture. (the first one, in 2001, not the second one from 2022). The two full papers [here] and [here] on determining the mechanism of the reaction are classic examples of meticulous, systematic experimental work.
Quiz Yourself!
Here’s a quiz on identifying the relationship between products of epoxidation reactions. (Note – for more examples of these types of quizzes, see this post on “What’s A Racemic Mixture“.)
Become a MOC member to see the clickable quiz with answers on the back.
Become a MOC member to see the clickable quiz with answers on the back.
Become a MOC member to see the clickable quiz with answers on the back.
Become a MOC member to see the clickable quiz with answers on the back.
Become a MOC member to see the clickable quiz with answers on the back.
(Advanced) References and Further Reading
(Advanced) References and Further Reading
- Oxydation ungesättigter Verbindungen mittels organischer Superoxyde
Nikolaus Prileschaev
Chem. Ber. 1909, 42, 4811.
DOI: 10.1002/cber.190904204100
This reaction (epoxidations of alkenes with a peracid) is also known as the Prizelhaev reaction after the author. - The oxidation of olefins with perbenzoic acids. A kinetic study
M. Lynch and K. H. Pausacker.
J. Chem. Soc. 1955, 1525-1531.
DOI: 10.1039/JR9550001525
One of the earliest papers on epoxidation with m-CPBA, comparing its reactivity with other substituted peracids. As expected, the reactivity of peroxyacids is increased by electron-withdrawing groups. - m-CHLOROPERBENZOIC ACID
Richard N. McDonald, Richard N. Steppel, and James E. Dorsey.
Org. Synth. 1970, 50, 15.
DOI: 10.15227/orgsyn.050.0015
A reliable preparation for m-CPBA (which is commercially available) in Organic Syntheses. As this procedure shows, m-CPBA is not prepared as a pure compound (it is a mixture of the peracid and acid, and commercial samples may contain residual water for stability). - Epoxidations with m-Chloroperbenzoic Acid
Nelson N. Schwartz and John H. Blumbergs.
J. Org. Chem. 1964 29, (7), 1976-1979.
DOI: 1021/jo01030a078
This paper describes mechanistic studies of m-CPBA oxidation that demonstrate that ionic intermediates are not involved in the reaction, and that the rate is insensitive to solvent polarity. - Record of chemical progress
Bartlett, P. D.
Chem. Prog. 1950, 11, 47
[Link]
This is the publication in which Prof. P. D. Bartlett describes the ‘butterfly mechanism’ for m-CPBA epoxidation. - MCPBA Epoxidation of Alkenes: Reinvestigation of Correlation between Rate and Ionization Potential
Cheal Kim, Teddy G. Traylor, and Charles L. Perrin.
J. Am. Chem. Soc. 1998, 120, (37), 9513-9516.
DOI: 1021/ja981531e
An interesting paper that describes the development of a kinetic method for measuring the rate of epoxidation of various alkenes with m-CPBA. - Experimental Geometry of the Epoxidation Transition State
Daniel A. Singleton, Steven R. Merrigan, Jian Liu, and K. N. Houk.
J. Am. Chem. Soc. 1997, 119, (14), 3385-3386.
DOI: 1021/ja963656u
Combined experimental and theoretical studies of the epoxidation transition state, showing that both C-O bond forming events are nearly synchronous. - The mechanism of epoxidation of olefins by peracids
V. G. Dryuk.
Tetrahedron. Volume 32, Issue 23, 1976, 2855-2866.
DOI:10.1016/0040-4020(76)80137-8
An account of the author’s work on kinetic studies of the epoxidation of olefins with peracids in order to determine the exact mechanism. - The Bond Dissociation Energy of Peroxides Revisited
https://pubs.acs.org/action/showCitFormats?doi=10.1021/acs.jpca.0c02859&ref=pdf - The first practical method for asymmetric epoxidation
Tsutomu Katsuki and K. Barry Sharpless
Journal of the American Chemical Society 1980 102 (18), 5974-5976
DOI: 10.1021/ja00538a077
Katsuki and Sharpless’ first report from 1980 on the asymmetric epoxidation reaction of allylic alcohols.
00 General Chemistry Review
01 Bonding, Structure, and Resonance
- How Do We Know Methane (CH4) Is Tetrahedral?
- Hybrid Orbitals and Hybridization
- How To Determine Hybridization: A Shortcut
- Orbital Hybridization And Bond Strengths
- Sigma bonds come in six varieties: Pi bonds come in one
- A Key Skill: How to Calculate Formal Charge
- The Four Intermolecular Forces and How They Affect Boiling Points
- 3 Trends That Affect Boiling Points
- How To Use Electronegativity To Determine Electron Density (and why NOT to trust formal charge)
- Introduction to Resonance
- How To Use Curved Arrows To Interchange Resonance Forms
- Evaluating Resonance Forms (1) - The Rule of Least Charges
- How To Find The Best Resonance Structure By Applying Electronegativity
- Evaluating Resonance Structures With Negative Charges
- Evaluating Resonance Structures With Positive Charge
- Exploring Resonance: Pi-Donation
- Exploring Resonance: Pi-acceptors
- In Summary: Evaluating Resonance Structures
- Drawing Resonance Structures: 3 Common Mistakes To Avoid
- How to apply electronegativity and resonance to understand reactivity
- Bond Hybridization Practice
- Structure and Bonding Practice Quizzes
- Resonance Structures Practice
02 Acid Base Reactions
- Introduction to Acid-Base Reactions
- Acid Base Reactions In Organic Chemistry
- The Stronger The Acid, The Weaker The Conjugate Base
- Walkthrough of Acid-Base Reactions (3) - Acidity Trends
- Five Key Factors That Influence Acidity
- Acid-Base Reactions: Introducing Ka and pKa
- How to Use a pKa Table
- The pKa Table Is Your Friend
- A Handy Rule of Thumb for Acid-Base Reactions
- Acid Base Reactions Are Fast
- pKa Values Span 60 Orders Of Magnitude
- How Protonation and Deprotonation Affect Reactivity
- Acid Base Practice Problems
03 Alkanes and Nomenclature
- Meet the (Most Important) Functional Groups
- Condensed Formulas: Deciphering What the Brackets Mean
- Hidden Hydrogens, Hidden Lone Pairs, Hidden Counterions
- Don't Be Futyl, Learn The Butyls
- Primary, Secondary, Tertiary, Quaternary In Organic Chemistry
- Branching, and Its Affect On Melting and Boiling Points
- The Many, Many Ways of Drawing Butane
- Wedge And Dash Convention For Tetrahedral Carbon
- Common Mistakes in Organic Chemistry: Pentavalent Carbon
- Table of Functional Group Priorities for Nomenclature
- Summary Sheet - Alkane Nomenclature
- Organic Chemistry IUPAC Nomenclature Demystified With A Simple Puzzle Piece Approach
- Boiling Point Quizzes
- Organic Chemistry Nomenclature Quizzes
04 Conformations and Cycloalkanes
- Staggered vs Eclipsed Conformations of Ethane
- Conformational Isomers of Propane
- Newman Projection of Butane (and Gauche Conformation)
- Introduction to Cycloalkanes
- Geometric Isomers In Small Rings: Cis And Trans Cycloalkanes
- Calculation of Ring Strain In Cycloalkanes
- Cycloalkanes - Ring Strain In Cyclopropane And Cyclobutane
- Cyclohexane Conformations
- Cyclohexane Chair Conformation: An Aerial Tour
- How To Draw The Cyclohexane Chair Conformation
- The Cyclohexane Chair Flip
- The Cyclohexane Chair Flip - Energy Diagram
- Substituted Cyclohexanes - Axial vs Equatorial
- Ranking The Bulkiness Of Substituents On Cyclohexanes: "A-Values"
- Cyclohexane Chair Conformation Stability: Which One Is Lower Energy?
- Fused Rings - Cis-Decalin and Trans-Decalin
- Naming Bicyclic Compounds - Fused, Bridged, and Spiro
- Bredt's Rule (And Summary of Cycloalkanes)
- Newman Projection Practice
- Cycloalkanes Practice Problems
05 A Primer On Organic Reactions
- The Most Important Question To Ask When Learning a New Reaction
- Curved Arrows (for reactions)
- Nucleophiles and Electrophiles
- The Three Classes of Nucleophiles
- Nucleophilicity vs. Basicity
- What Makes A Good Nucleophile?
- What Makes A Good Leaving Group?
- 3 Factors That Stabilize Carbocations
- Equilibrium and Energy Relationships
- 7 Factors that stabilize negative charge in organic chemistry
- 7 Factors That Stabilize Positive Charge in Organic Chemistry
- What's a Transition State?
- Hammond's Postulate
- Learning Organic Chemistry Reactions: A Checklist (PDF)
- Introduction to Oxidative Cleavage Reactions
06 Free Radical Reactions
- Bond Dissociation Energies = Homolytic Cleavage
- Free Radical Reactions
- 3 Factors That Stabilize Free Radicals
- What Factors Destabilize Free Radicals?
- Bond Strengths And Radical Stability
- Free Radical Initiation: Why Is "Light" Or "Heat" Required?
- Initiation, Propagation, Termination
- Monochlorination Products Of Propane, Pentane, And Other Alkanes
- Selectivity In Free Radical Reactions
- Selectivity in Free Radical Reactions: Bromination vs. Chlorination
- Halogenation At Tiffany's
- Allylic Bromination
- Bonus Topic: Allylic Rearrangements
- In Summary: Free Radicals
- Synthesis (2) - Reactions of Alkanes
- Free Radicals Practice Quizzes
07 Stereochemistry and Chirality
- Types of Isomers: Constitutional Isomers, Stereoisomers, Enantiomers, and Diastereomers
- How To Draw The Enantiomer Of A Chiral Molecule
- How To Draw A Bond Rotation
- Introduction to Assigning (R) and (S): The Cahn-Ingold-Prelog Rules
- Assigning Cahn-Ingold-Prelog (CIP) Priorities (2) - The Method of Dots
- Enantiomers vs Diastereomers vs The Same? Two Methods For Solving Problems
- Assigning R/S To Newman Projections (And Converting Newman To Line Diagrams)
- How To Determine R and S Configurations On A Fischer Projection
- The Meso Trap
- Optical Rotation, Optical Activity, and Specific Rotation
- Optical Purity and Enantiomeric Excess
- What's a Racemic Mixture?
- Chiral Allenes And Chiral Axes
- Stereochemistry Practice Problems and Quizzes
08 Substitution Reactions
- Nucleophilic Substitution Reactions - Introduction
- Two Types of Nucleophilic Substitution Reactions
- The SN2 Mechanism
- Why the SN2 Reaction Is Powerful
- The SN1 Mechanism
- The Conjugate Acid Is A Better Leaving Group
- Comparing the SN1 and SN2 Reactions
- Polar Protic? Polar Aprotic? Nonpolar? All About Solvents
- Steric Hindrance is Like a Fat Goalie
- Common Blind Spot: Intramolecular Reactions
- Substitution Practice - SN1
- Substitution Practice - SN2
09 Elimination Reactions
- Elimination Reactions (1): Introduction And The Key Pattern
- Elimination Reactions (2): The Zaitsev Rule
- Elimination Reactions Are Favored By Heat
- Two Elimination Reaction Patterns
- The E1 Reaction
- The E2 Mechanism
- E1 vs E2: Comparing the E1 and E2 Reactions
- Antiperiplanar Relationships: The E2 Reaction and Cyclohexane Rings
- Bulky Bases in Elimination Reactions
- Comparing the E1 vs SN1 Reactions
- Elimination (E1) Reactions With Rearrangements
- E1cB - Elimination (Unimolecular) Conjugate Base
- Elimination (E1) Practice Problems And Solutions
- Elimination (E2) Practice Problems and Solutions
10 Rearrangements
11 SN1/SN2/E1/E2 Decision
- Identifying Where Substitution and Elimination Reactions Happen
- Deciding SN1/SN2/E1/E2 (1) - The Substrate
- Deciding SN1/SN2/E1/E2 (2) - The Nucleophile/Base
- SN1 vs E1 and SN2 vs E2 : The Temperature
- Deciding SN1/SN2/E1/E2 - The Solvent
- Wrapup: The Key Factors For Determining SN1/SN2/E1/E2
- Alkyl Halide Reaction Map And Summary
- SN1 SN2 E1 E2 Practice Problems
12 Alkene Reactions
- E and Z Notation For Alkenes (+ Cis/Trans)
- Alkene Stability
- Alkene Addition Reactions: "Regioselectivity" and "Stereoselectivity" (Syn/Anti)
- Stereoselective and Stereospecific Reactions
- Hydrohalogenation of Alkenes and Markovnikov's Rule
- Hydration of Alkenes With Aqueous Acid
- Rearrangements in Alkene Addition Reactions
- Halogenation of Alkenes and Halohydrin Formation
- Oxymercuration Demercuration of Alkenes
- Hydroboration Oxidation of Alkenes
- m-CPBA (meta-chloroperoxybenzoic acid)
- OsO4 (Osmium Tetroxide) for Dihydroxylation of Alkenes
- Palladium on Carbon (Pd/C) for Catalytic Hydrogenation of Alkenes
- Cyclopropanation of Alkenes
- A Fourth Alkene Addition Pattern - Free Radical Addition
- Alkene Reactions: Ozonolysis
- Summary: Three Key Families Of Alkene Reaction Mechanisms
- Synthesis (4) - Alkene Reaction Map, Including Alkyl Halide Reactions
- Alkene Reactions Practice Problems
13 Alkyne Reactions
- Acetylides from Alkynes, And Substitution Reactions of Acetylides
- Partial Reduction of Alkynes With Lindlar's Catalyst
- Partial Reduction of Alkynes With Na/NH3 To Obtain Trans Alkenes
- Alkyne Hydroboration With "R2BH"
- Hydration and Oxymercuration of Alkynes
- Hydrohalogenation of Alkynes
- Alkyne Halogenation: Bromination and Chlorination of Alkynes
- Alkyne Reactions - The "Concerted" Pathway
- Alkenes To Alkynes Via Halogenation And Elimination Reactions
- Alkynes Are A Blank Canvas
- Synthesis (5) - Reactions of Alkynes
- Alkyne Reactions Practice Problems With Answers
14 Alcohols, Epoxides and Ethers
- Alcohols - Nomenclature and Properties
- Alcohols Can Act As Acids Or Bases (And Why It Matters)
- Alcohols - Acidity and Basicity
- The Williamson Ether Synthesis
- Ethers From Alkenes, Tertiary Alkyl Halides and Alkoxymercuration
- Alcohols To Ethers via Acid Catalysis
- Cleavage Of Ethers With Acid
- Epoxides - The Outlier Of The Ether Family
- Opening of Epoxides With Acid
- Epoxide Ring Opening With Base
- Making Alkyl Halides From Alcohols
- Tosylates And Mesylates
- PBr3 and SOCl2
- Elimination Reactions of Alcohols
- Elimination of Alcohols To Alkenes With POCl3
- Alcohol Oxidation: "Strong" and "Weak" Oxidants
- Demystifying The Mechanisms of Alcohol Oxidations
- Protecting Groups For Alcohols
- Thiols And Thioethers
- Calculating the oxidation state of a carbon
- Oxidation and Reduction in Organic Chemistry
- Oxidation Ladders
- SOCl2 Mechanism For Alcohols To Alkyl Halides: SN2 versus SNi
- Alcohol Reactions Roadmap (PDF)
- Alcohol Reaction Practice Problems
- Epoxide Reaction Quizzes
- Oxidation and Reduction Practice Quizzes
15 Organometallics
- What's An Organometallic?
- Formation of Grignard and Organolithium Reagents
- Organometallics Are Strong Bases
- Reactions of Grignard Reagents
- Protecting Groups In Grignard Reactions
- Synthesis Problems Involving Grignard Reagents
- Grignard Reactions And Synthesis (2)
- Organocuprates (Gilman Reagents): How They're Made
- Gilman Reagents (Organocuprates): What They're Used For
- The Heck, Suzuki, and Olefin Metathesis Reactions (And Why They Don't Belong In Most Introductory Organic Chemistry Courses)
- Reaction Map: Reactions of Organometallics
- Grignard Practice Problems
16 Spectroscopy
- Degrees of Unsaturation (or IHD, Index of Hydrogen Deficiency)
- Conjugation And Color (+ How Bleach Works)
- Introduction To UV-Vis Spectroscopy
- UV-Vis Spectroscopy: Absorbance of Carbonyls
- UV-Vis Spectroscopy: Practice Questions
- Bond Vibrations, Infrared Spectroscopy, and the "Ball and Spring" Model
- Infrared Spectroscopy: A Quick Primer On Interpreting Spectra
- IR Spectroscopy: 4 Practice Problems
- 1H NMR: How Many Signals?
- Homotopic, Enantiotopic, Diastereotopic
- Diastereotopic Protons in 1H NMR Spectroscopy: Examples
- 13-C NMR - How Many Signals
- Liquid Gold: Pheromones In Doe Urine
- Natural Product Isolation (1) - Extraction
- Natural Product Isolation (2) - Purification Techniques, An Overview
- Structure Determination Case Study: Deer Tarsal Gland Pheromone
17 Dienes and MO Theory
- What To Expect In Organic Chemistry 2
- Are these molecules conjugated?
- Conjugation And Resonance In Organic Chemistry
- Bonding And Antibonding Pi Orbitals
- Molecular Orbitals of The Allyl Cation, Allyl Radical, and Allyl Anion
- Pi Molecular Orbitals of Butadiene
- Reactions of Dienes: 1,2 and 1,4 Addition
- Thermodynamic and Kinetic Products
- More On 1,2 and 1,4 Additions To Dienes
- s-cis and s-trans
- The Diels-Alder Reaction
- Cyclic Dienes and Dienophiles in the Diels-Alder Reaction
- Stereochemistry of the Diels-Alder Reaction
- Exo vs Endo Products In The Diels Alder: How To Tell Them Apart
- HOMO and LUMO In the Diels Alder Reaction
- Why Are Endo vs Exo Products Favored in the Diels-Alder Reaction?
- Diels-Alder Reaction: Kinetic and Thermodynamic Control
- The Retro Diels-Alder Reaction
- The Intramolecular Diels Alder Reaction
- Regiochemistry In The Diels-Alder Reaction
- The Cope and Claisen Rearrangements
- Electrocyclic Reactions
- Electrocyclic Ring Opening And Closure (2) - Six (or Eight) Pi Electrons
- Diels Alder Practice Problems
- Molecular Orbital Theory Practice
18 Aromaticity
- Introduction To Aromaticity
- Rules For Aromaticity
- Huckel's Rule: What Does 4n+2 Mean?
- Aromatic, Non-Aromatic, or Antiaromatic? Some Practice Problems
- Antiaromatic Compounds and Antiaromaticity
- The Pi Molecular Orbitals of Benzene
- The Pi Molecular Orbitals of Cyclobutadiene
- Frost Circles
- Aromaticity Practice Quizzes
19 Reactions of Aromatic Molecules
- Electrophilic Aromatic Substitution: Introduction
- Activating and Deactivating Groups In Electrophilic Aromatic Substitution
- Electrophilic Aromatic Substitution - The Mechanism
- Ortho-, Para- and Meta- Directors in Electrophilic Aromatic Substitution
- Understanding Ortho, Para, and Meta Directors
- Why are halogens ortho- para- directors?
- Disubstituted Benzenes: The Strongest Electron-Donor "Wins"
- Electrophilic Aromatic Substitutions (1) - Halogenation of Benzene
- Electrophilic Aromatic Substitutions (2) - Nitration and Sulfonation
- EAS Reactions (3) - Friedel-Crafts Acylation and Friedel-Crafts Alkylation
- Intramolecular Friedel-Crafts Reactions
- Nucleophilic Aromatic Substitution (NAS)
- Nucleophilic Aromatic Substitution (2) - The Benzyne Mechanism
- Reactions on the "Benzylic" Carbon: Bromination And Oxidation
- The Wolff-Kishner, Clemmensen, And Other Carbonyl Reductions
- More Reactions on the Aromatic Sidechain: Reduction of Nitro Groups and the Baeyer Villiger
- Aromatic Synthesis (1) - "Order Of Operations"
- Synthesis of Benzene Derivatives (2) - Polarity Reversal
- Aromatic Synthesis (3) - Sulfonyl Blocking Groups
- Birch Reduction
- Synthesis (7): Reaction Map of Benzene and Related Aromatic Compounds
- Aromatic Reactions and Synthesis Practice
- Electrophilic Aromatic Substitution Practice Problems
20 Aldehydes and Ketones
- What's The Alpha Carbon In Carbonyl Compounds?
- Nucleophilic Addition To Carbonyls
- Aldehydes and Ketones: 14 Reactions With The Same Mechanism
- Sodium Borohydride (NaBH4) Reduction of Aldehydes and Ketones
- Grignard Reagents For Addition To Aldehydes and Ketones
- Wittig Reaction
- Hydrates, Hemiacetals, and Acetals
- Imines - Properties, Formation, Reactions, and Mechanisms
- All About Enamines
- Breaking Down Carbonyl Reaction Mechanisms: Reactions of Anionic Nucleophiles (Part 2)
- Aldehydes Ketones Reaction Practice
21 Carboxylic Acid Derivatives
- Nucleophilic Acyl Substitution (With Negatively Charged Nucleophiles)
- Addition-Elimination Mechanisms With Neutral Nucleophiles (Including Acid Catalysis)
- Basic Hydrolysis of Esters - Saponification
- Transesterification
- Proton Transfer
- Fischer Esterification - Carboxylic Acid to Ester Under Acidic Conditions
- Lithium Aluminum Hydride (LiAlH4) For Reduction of Carboxylic Acid Derivatives
- LiAlH[Ot-Bu]3 For The Reduction of Acid Halides To Aldehydes
- Di-isobutyl Aluminum Hydride (DIBAL) For The Partial Reduction of Esters and Nitriles
- Amide Hydrolysis
- Thionyl Chloride (SOCl2) And Conversion of Carboxylic Acids to Acid Halides
- Diazomethane (CH2N2)
- Carbonyl Chemistry: Learn Six Mechanisms For the Price Of One
- Making Music With Mechanisms (PADPED)
- Carboxylic Acid Derivatives Practice Questions
22 Enols and Enolates
- Keto-Enol Tautomerism
- Enolates - Formation, Stability, and Simple Reactions
- Kinetic Versus Thermodynamic Enolates
- Aldol Addition and Condensation Reactions
- Reactions of Enols - Acid-Catalyzed Aldol, Halogenation, and Mannich Reactions
- Claisen Condensation and Dieckmann Condensation
- Decarboxylation
- The Malonic Ester and Acetoacetic Ester Synthesis
- The Michael Addition Reaction and Conjugate Addition
- The Robinson Annulation
- Haloform Reaction
- The Hell–Volhard–Zelinsky Reaction
- Enols and Enolates Practice Quizzes
23 Amines
- The Amide Functional Group: Properties, Synthesis, and Nomenclature
- Basicity of Amines And pKaH
- 5 Key Basicity Trends of Amines
- The Mesomeric Effect And Aromatic Amines
- Nucleophilicity of Amines
- Alkylation of Amines (Sucks!)
- Reductive Amination
- The Gabriel Synthesis
- Some Reactions of Azides
- The Hofmann Elimination
- The Hofmann and Curtius Rearrangements
- The Cope Elimination
- Protecting Groups for Amines - Carbamates
- The Strecker Synthesis of Amino Acids
- Introduction to Peptide Synthesis
- Reactions of Diazonium Salts: Sandmeyer and Related Reactions
- Amine Practice Questions
24 Carbohydrates
- D and L Notation For Sugars
- Pyranoses and Furanoses: Ring-Chain Tautomerism In Sugars
- What is Mutarotation?
- Reducing Sugars
- The Big Damn Post Of Carbohydrate-Related Chemistry Definitions
- The Haworth Projection
- Converting a Fischer Projection To A Haworth (And Vice Versa)
- Reactions of Sugars: Glycosylation and Protection
- The Ruff Degradation and Kiliani-Fischer Synthesis
- Isoelectric Points of Amino Acids (and How To Calculate Them)
- Carbohydrates Practice
- Amino Acid Quizzes
25 Fun and Miscellaneous
- A Gallery of Some Interesting Molecules From Nature
- Screw Organic Chemistry, I'm Just Going To Write About Cats
- On Cats, Part 1: Conformations and Configurations
- On Cats, Part 2: Cat Line Diagrams
- On Cats, Part 4: Enantiocats
- On Cats, Part 6: Stereocenters
- Organic Chemistry Is Shit
- The Organic Chemistry Behind "The Pill"
- Maybe they should call them, "Formal Wins" ?
- Why Do Organic Chemists Use Kilocalories?
- The Principle of Least Effort
- Organic Chemistry GIFS - Resonance Forms
- Reproducibility In Organic Chemistry
- What Holds The Nucleus Together?
- How Reactions Are Like Music
- Organic Chemistry and the New MCAT
26 Organic Chemistry Tips and Tricks
- Common Mistakes: Formal Charges Can Mislead
- Partial Charges Give Clues About Electron Flow
- Draw The Ugly Version First
- Organic Chemistry Study Tips: Learn the Trends
- The 8 Types of Arrows In Organic Chemistry, Explained
- Top 10 Skills To Master Before An Organic Chemistry 2 Final
- Common Mistakes with Carbonyls: Carboxylic Acids... Are Acids!
- Planning Organic Synthesis With "Reaction Maps"
- Alkene Addition Pattern #1: The "Carbocation Pathway"
- Alkene Addition Pattern #2: The "Three-Membered Ring" Pathway
- Alkene Addition Pattern #3: The "Concerted" Pathway
- Number Your Carbons!
- The 4 Major Classes of Reactions in Org 1
- How (and why) electrons flow
- Grossman's Rule
- Three Exam Tips
- A 3-Step Method For Thinking Through Synthesis Problems
- Putting It Together
- Putting Diels-Alder Products in Perspective
- The Ups and Downs of Cyclohexanes
- The Most Annoying Exceptions in Org 1 (Part 1)
- The Most Annoying Exceptions in Org 1 (Part 2)
- The Marriage May Be Bad, But the Divorce Still Costs Money
- 9 Nomenclature Conventions To Know
- Nucleophile attacks Electrophile
27 Case Studies of Successful O-Chem Students
- Success Stories: How Corina Got The The "Hard" Professor - And Got An A+ Anyway
- How Helena Aced Organic Chemistry
- From a "Drop" To B+ in Org 2 – How A Hard Working Student Turned It Around
- How Serge Aced Organic Chemistry
- Success Stories: How Zach Aced Organic Chemistry 1
- Success Stories: How Kari Went From C– to B+
- How Esther Bounced Back From a "C" To Get A's In Organic Chemistry 1 And 2
- How Tyrell Got The Highest Grade In Her Organic Chemistry Course
- This Is Why Students Use Flashcards
- Success Stories: How Stu Aced Organic Chemistry
- How John Pulled Up His Organic Chemistry Exam Grades
- Success Stories: How Nathan Aced Organic Chemistry (Without It Taking Over His Life)
- How Chris Aced Org 1 and Org 2
- Interview: How Jay Got an A+ In Organic Chemistry
- How to Do Well in Organic Chemistry: One Student's Advice
- "America's Top TA" Shares His Secrets For Teaching O-Chem
- "Organic Chemistry Is Like..." - A Few Metaphors
- How To Do Well In Organic Chemistry: Advice From A Tutor
- Guest post: "I went from being afraid of tests to actually looking forward to them".
For the epoxidation of the cis alkene, would product (B) also form as a mixture of enantiomers? Thank you for a wonderful site.
Yes – it will, sorry, I should have put that in there!
for 1,3-cyclohexadiene reacts with peroxyacetic acid to form epoxide, there is very small amount of diepoxide formed (further epoxidation of monoepoxide). What’s the reason for that?
Well, after one epoxidation of the diene you’ll still have an alkene. It won’t be as reactive as the diene, but it is still capable of undergoing epoxidation.
electron donating group increase the rate of epoxidation of an alkene with perbenzoic acid. explain
mCPBA is the electrophile in this reaction and the alkene is the nucleophile. In the transition state, the C-C pi bond is breaking and there are partial positive charges on the alkenyl carbons. So groups bonded to the alkenyl group that help to stabilize the positive charges will lead to a more stable (lower energy) transition state, accelerating the reaction.
Then what if there is ketone with vinyl group like ‘1-phenylbut-3-en-1-one’, is there epoxidation or electrophilic addition?
Epoxidation won’t happen except with electron-rich alkenes. The most likely outcome is a Baeyer-Villiger.
How to quench mcbpa if sm material is acid and product also acid
Just quench with bisulfite to get rid of the oxidant, acidify and extract. You can run columns on carboxylic acids if you acidify your eluent.
Why does m-CPBA acts as a electrophile in epoxidation reaction?
The O-O bond is quite weak (about 35 kcal/mol) and the carboxylate is a good leaving group. So nucleophilic attack at the terminal oxygen is quite easy.
Hi!!! Is mCPBA able to react with alkynes? I have a feeling no but I am not 100% sure (I want to make sure that I don’t get this wrong on an exam later).
Funny you should ask, I was very close to putting something up about this! For your purposes, I would say, “No”, as the reaction between alkynes and mCBA is not very useful.
However… the reaction between alkynes and mCPBA that *does* happen is quite a trip. I can say with 99.9% certainty it will not show up on an exam. https://imgur.com/a/Ibvqy8y
What happend when a compound on reaction with mcpba which has both doble bond and a ketonic moeity
It depends! I once used mCPBA in a reaction which had a diene and two ketones. The diene was electron poor (connected to an ester) and only one of the ketones was really accessible. So it did a Baeyer-Villiger!
Hello,
I have a question about epoxy.
i want to form a epoxy while i have on the left side an alcohol and on the right side a dubble bound. so for an example [(2R,3S)-3-Allyl-2-oxrianyl]methanol. can someone help me? because i know how to form the left side but not the right side.
Sounds like glycidol. You can buy that.
https://www.google.com/search?q=glycidol&rlz=1C5CHFA_enUS503US504&oq=glycidol&aqs=chrome..69i57j0l7.1372j0j4&sourceid=chrome&ie=UTF-8
Which o in mcpba the is electron deficient and y electron defficient plzz ans
Answer me this: What’s a better leaving group: R-CO2(-) or HO(-) ? Therein lies your answer.
I understand that the reaction of peroxy acid and styrene will give me epoxystyrene. However, there
is a by product in about 25 percent yield which is benzoic acid. What is the mechanism for this reaction.
Are you sure it’s not a byproduct from the peroxyacid?
Styrene + perbenzoic acid gives great yields of styrene oxide. See:
http://www.orgsyn.org/demo.aspx?prep=CV1P0494
What if the ketone is cyclic, like 4-methyl cyclohexanone? Does the ring break?
Great question! You form a lactone – a cyclic ester – in that case a 7-membered ring.
does the reaction vary when mCPBA is in acidic or basic solvent or does it strictly depend on the reactant being cis/trans
The epoxidation reaction is stereospecific. Under identical conditions, epoxidation of a cis alkene and a trans alkene will give two stereoisomeric products. This is true whether mCPBA is used in its acidic form, or if a mild base (e.g. NaHCO3) is used with mCPBA to promote the reaction.
Why does the oxygen with the hydrogen function as a nucleophile and not the other oxygen?
Because after the initial nucleophilic attack, the oxygen with the hydrogen can be quickly neutralized by removal of a proton, whereas the “other oxygen” cannot go back to being a neutral species without breaking C-O or O-O. Try and draw out the arrows for a reaction with that oxygen.
Do migrating aptitude of phenyl greater than H in baeyer villager oxidation .. ?
If yes then ph-CHO with per acid will not give ph-COOH?.. Please reply
No, H has the best migratory aptitude. BV oxidation of an aldehyde will give a carboxylic acid.
can epoxides exhibit geometrical isomerism??/
The two C-O bonds to the epoxide will always be on the same face.
Why is the oxygen oxygen bond in per acid is too weak??
The oxygen-oxygen bond is weak due to repulsion between the lone pairs residing on the adjacent oxygens.
Hi, I have a question,
For my masters project the mCPBA didn’t work to epoxides my olefin, how can I explain this? (it was an epoxidation of an allyl ether)
And are there any references I could use to explain this mechanism?
Thank you :)
It’s hard to answer a general question like this without more specifics. There are lots of reasons why it might not work, such as the quality of the reagent, the electron density of the alkene, steric effects around the alkene, etc.
Why only meta Cpba is used. Why not the para Cpba is used for the oxidation?
The meta isomer is used because it’s cheap. It’s easier to prepare from chlorination of (cheap) benzoic acid, since the carboxylic acid is a meta-directing group. Adding electron withdrawing groups on to the aromatic ring would make it more reactive.
What if there’s two double bonds? Would the one that has more aklye halides get priority?
The double bond attached to more alkyl groups (or fewer hydrogens, if you prefer) gets priority, as it would be more electron-rich.
This seems a little odd to me because if you look at the stability of products, the one with note hyperconjugation would be more stable, which in the case dec mentioned would mean that the double bond attached to less alkyl groups would be preferred?
Is this an electrophilic addition reaction
Yes, it is an addition reaction, and the reagent (mCPBA) is an electrophile. So this would qualify.