Dienes and MO Theory
Electrocyclic Ring Opening And Closure (2) – Six (or Eight) Pi Electrons
Last updated: October 27th, 2022 |
Electrocyclic Ring Opening And Closure (2) – Systems With 6 (and 8) Pi-Electrons.
In the last post we introduced electrocyclic ring opening and closure, with conjugated systems of two pi bonds (4 pi electrons). [See Post: Electrocyclic Reactions] . We saw that systems with 4pi electrons undergo “conrotatory” ring closure (and opening) under thermal conditions, and “disrotatory” ring closure (and opening) under photochemical conditions. [We’ll re-introduce those terms in the article below]
So what happens if we add an extra pi bond to the system, giving us 6 pi-electrons? How does this change things?
In case you want to skip right to the answer, here’s the short summary.
Electrocyclic ring-closing and ring opening in 6 pi systems occur in exactly the opposite sense of those in 4 pi systems.
Why is that, you might ask? Great question! In the article below, we’ll go through this subject from the beginning.
Table of Contents
- 6 pi Thermal Electrocyclic Ring Opening and Closing
- Going “Under The Hood” of 6-π Electrocyclic Ring Closure: Identifying The Highest-Occupied Molecular Orbital (HOMO)
- Disrotatory and Conrotatory Ring Closure: The Importance of “Orbital Symmetry”
- What About Electrocyclic Ring Opening?
- Electrocyclic Reactions Are Stereospecific
- Electrocyclic Ring Closure (And Opening) Under “Photochemical” Conditions
- Summary: 6 pi Electrocyclic Ring Opening and Closure
- [Bonus] What About 8 pi Systems?
- Notes
- Quiz Yourself!
- (Advanced) References and Further Reading
1. 6 pi Thermal Electrocyclic Ring Opening and Closing
So when we say, a “4 pi” , “6 pi”, or even “n-pi” system, what’s meant by that?
What we mean is a system of 4, 6 or even “n” atoms bearing p-orbitals able to overlap (“conjugate”) such that a set of 4, 6, or “n” pi molecular orbitals is formed. [See: Conjugation and Resonance]. Since each pi-bond is comprised of two adjacent p orbitals, a molecule with three conjugated pi bonds results in a “pi system” containing 6 pi molecular orbitals.
Let’s start with the simplest possible 6 pi example that can undergo electrocyclic ring closure: (Z)- hexatri-1,3,5-ene. When this triene is heated, a new species is formed that looks like this:
[Note: this wouldn’t this work for the E isomer. Why not? [See Note 1]
Note the bonds that form and break here. We’re breaking 3 C-C pi bonds and forming two C-C pi bonds along with a sigma bond.
The reverse of electrocyclic ring-closure is electrocyclic ring-opening. . Heating 1,3-cyclohexadiene will result in at least some (Z)- hexa-1,3,5-triene:
Note that the bonds that form and break here are the exact opposite than those found in the forward reaction.
Both electrocyclic ring-opening and ring-closure proceed in a concerted fashion (with no intermediate) and pass through a common transition state. These reactions belong to a class of concerted reactions with cyclic transition states known as pericyclic reactions, which includes the Diels-Alder, the Cope and Claisen rearrangements, and many others.
Since the reactions are reversible and pass through the same transition state, we can also draw it up as an equilibrium.
Note 2 about the equilibrium shown here.
2. Going “Under The Hood” of 6-π Electrocyclic Ring Closure: Identifying The Highest-Occupied Molecular Orbital (HOMO)
If you look at the bonds formed / bonds broken table, you’ll see that there’s a net destruction of one pi bond and net formation of one sigma bond.
So what’s actually going on when hexatriene is being converted to 1,3 cyclohexadiene?
Well, the new sigma bond is formed from the overlap of two p-orbitals as the ends of the hexatriene pi system (carbon 1 and carbon 6) rotate, and the hybridization of these carbons changes from sp2 to sp3 . (We’ll get to this in a second).
The pi molecular orbital involved in this transformation will be the one in which the electrons are the most loosely-held, or in other words, those of highest energy.
What are the highest-energy electrons in this case? The electrons in the highest-energy molecular orbital (HOMO for short).
So how do we know what the HOMO looks like? Glad you asked.
We covered how to build up pi molecular orbitals in this earlier post. [See: Pi molecular orbitals of butadiene]
The pi molecular orbitals of 1,3,5 hexatriene look like this:
That’s a lot to absorb! Thankfully, for our purposes, we don’t need to use all the molecular orbitals of 1,3,5 hexatriene in this instance.
What we really care about here is the highest-energy (most loosely-held) electrons; the electrons in the highest-occupied molecular orbital (HOMO). In hexatriene, that’s π3, which we’ll draw out below:
We can simplify things even further. For the purposes of forming the new sigma bond, we’re only going to concern ourselves with the orbitals on C1 and C6, since these are the orbitals involved in forming the new sigma bond.
Now we can map this on to 1,3,5 hexatriene drawn in the conformation where the reaction will occur.
Now what?
3. Disrotatory and Conrotatory Ring Closure: The Importance of “Orbital Symmetry”
In order for the new sigma bond to form, C1 and C6 must rotate such that there is constructive overlap orbitals. They must have the same phase.
In a 6 pi system like 1,3,5 hexatriene, the orbitals on each of the termini (C1 and C6) each have their phases aligned in the same direction (they are arbitrarily drawn with the “shaded” lobe pointing up, but it would work just as well if they were both drawn with the shaded lobes pointing down). [If you like, you can call this a “symmetric” orientation; the term “orbital symmetry” is generally used to refer to the relative orientation of these lobes.]
Sigma bond formation can thus be achieved if one carbon rotates clockwise and the other counter-clockwise. This is referred to as a disrotatory mode of bond formation.
What if they rotate in the same direction? Since the lobes are of opposite phase, this results in destructive orbital overlap and a sigma bond cannot be formed. This mode of bond rotation is called, “conrotatory“.
We saw in the previous post that 4 pi thermal ring closure and opening occurs in a conrotatory manner. If you look at the arrangement of orbitals on C1 and C4 in the HOMO of 1,3-butadiene, you can see that they have an opposite arrangement to those on C1 and C6 of the HOMO of 1,3,5-hexatriene (“antisymmetric”, if you want to use a more specific term).
To see the full mechanism, hover here or click this link.
So, to summarize, adding two p-orbitals to the pi system changes the mode of thermal electrocyclic ring-closing from conrotatory (4 pi ) to disrotatory (6 pi).
4. What About Electrocyclic Ring Opening?
Imagine making a movie of electrocyclic ring closure based on what we just saw.
Now imagine running that movie in reverse.
What I’ve just described – the reverse of electrocyclic closure – is electrocyclic ring opening.
Electrocyclic ring opening and electrocyclic ring closure share the same transition state, so everything that applies to electrocyclic ring closure in the forward direction applies equally to electrocyclic ring opening (the reverse direction).
Electrocyclic ring opening under thermal conditions for a 6 pi system must occur in such a way that the sigma bond ruptures so as to regenerate the HOMO (π 3) of 1,3,5 hexatriene. So it must also be disrotatory.
Note 4 that in this figure the blue hydrogens are shown rotating “outward”. Would it work if the blue hydrogens were rotating “inward” as well? Yes, since that still counts as “disrotatory”. For each disrotatory and conrotatory mode, there are generally two possibilities (inward/outward). In this article, for brevity, we’re only going to show one.
5. Electrocyclic Reactions Are Stereospecific
So far, we’ve discussed “conrotatory” and “disrotatory” but haven’t really described any situations where the stereochemistry would come into play.
We’re now ready to see what happens when there are substituents on the end carbons of a 6 pi system like 1,3,5 hexatriene.
Here are two examples from the chemistry literature [See here]. Try to confirm for yourself that these ring closures are indeed disrotatory.
See how flipping the geometry about one double bond from E to Z results in flipping the geometry of the product?
Electrocyclic reactions are yet another example of a stereospecific reaction. From IUPAC: A reaction is termed stereospecific if starting materials differing only in their configuration are converted into stereoisomeric products. That’s true here, since the starting materials and products are each stereoisomers (diastereomers) of each other.
Electrocyclic ring opening also occurs in the disrotatory mode:
Figuring out the mode of rotation (disrotatory or conrotatory) can take some time and practice. It’s helpful to imagine looking at the molecule from the front, and tracing the path of each substituent as they go from starting material to product. Some practice quizzes are included at the bottom of the post so you can gain some more experience with these types of questions.
6. Electrocyclic Ring Closure (And Opening) Under “Photochemical” Conditions
As we saw in the 4pi system, it’s also possible to induce electrocyclic ring opening and closure through the influence of ultraviolet light (hν ).
However, the mode of rotation is different. In the 6pi system, we saw (above) that heating the system resulted in disrotatory ring closure.
Under photochemical conditions, the mode of ring closure occurs in the opposite direction.
These reactions are example of conrotatory ring closure, where each carbon on the terminus rotates in the same direction.
Why might ultraviolet light affect these reactions?
Recall that in our post on UV-Vis spectroscopy [See: Introduction to UV-Vis Spectroscopy], we saw that UV (and visible) light can promote electrons in pi systems from their highest occupied molecular orbital (HOMO) to the lowest-unoccupied molecular orbital (LUMO).
In effect, for hexatriene, this would be a transition from pi3 (the HOMO) to pi4 (the LUMO) which effectively becomes the “new” HOMO.
That means that if we’re looking at an electrocyclic reaction under these conditions, we need to do the analysis based on π4 instead of π3.
And the orbital symmetry of π4 is different. Let’s overlay π4 on 1,3,5-hexatriene. Then we’re back to a situation where the orbitals on the termini are antisymmetric – just as in the thermal 4 pi case.
Therefore we should expect to (and do!) see conrotatory ring closure.
Photochemical electrocyclic ring opening in 6 pi systems is also observed, and is likewise conrotatory.
You’re probably familiar with one example of this reaction, even if you don’t know it.
At some point, your parents probably told you to play outside so you could “get your vitamin D”.
What your parents didn’t tell you was that you needed the UV light (290-330 nm) to activate a 6 pi conrotatory electrocyclic ring opening to convert 7-dehydrochloresterol into Previtamin D3:
I pass this on to you, so you can tell your future children what’s really going on when they play outside.
[Note 3 – there’s a second pericyclic reaction involved in the formation of Vitamin D3 from “Previtamin D3” but we’re not going to go there today. ]
7. Summary: 6 pi Electrocyclic Ring Opening and Closure
That’s probably enough for one article. We can summarize the key patterns in a nice little table.
From the Chem 206 notes of Prof. David Evans comes a useful concept called the “toggle” algorithm.
Since the mode of rotation “flips” when going from thermal to photochemical conditions, or from adding 2 p-orbitals to the pi system, you only need to remember one of these cases, and you can derive all the others by using the “toggle” algorithm.
8. [Encore] What About 8 pi Systems?
OK, let’s face it. You can’t get enough information about orbital symmetry. You’re addicted, and want to know more.
I understand.
Is there more? Oh hell yes there is more, but we’ll just give one more example.
Since we’ve gone through 4pi (thermal and photochemical) and 6 pi (thermal and photochemical) you might have an urgent question on your mind: what about 8 pi systems?
Good news. If you know the “toggle algorithm” you can figure out the modes for both the thermal case and the photochemical case.
- Since 4 pi (thermal) is conrotatory, 6 pi (thermal) is disrotatory, one more toggle (8 pi) will move us back to conrotatory.
- Likewise, 4 pi (photochemical) is disrotatory, 6 pi (photochemical) is conrotatory, and one more toggle (8 pi) moves us back to disrotatory.
Electrocyclic reactions can also occur in sequence. For example, here is an 8 pi thermal electrocyclic ring closure (conrotatory) followed by a 6 pi thermal electrocyclic ring closure (disrotatory).
What’s even more interesting is that these reactions actually occur in nature! One of the most fascinating natural product syntheses of all time was the synthesis of the endiandric acids by the lab of K. C. Nicolaou, which has a rich feast of electrocyclic reactions to chew on. See references here. A very rewarding chapter in Classics in Total Synthesis also.
The synthesis is also covered on Hans Reich’s page, here: Endiandric Acids
Notes
Note 1. If the C3-C4 double bond was trans, the two termini wouldn’t meet.
Note 2. I’ve drawn the equilibrium arrows as “equal” here, but that shouldn’t be meant to imply that the equilibrium constant is 1. Generally, the ring closure should be favored over ring opening since C-C sigma bonds (about 80 kcal/mol) are stronger than C-C pi bonds (about 60 kcal/mol).
Note 3. “Pre-Vitamin D3” is converted into Vitamin D3 through an antarafacial 1,7 hydrogen shift, which we might get into in a later post. This site covers the topic nicely.
Note 4. In the interest of space, we didn’t really go into the fact that each mode (“conrotatory” and “disrotatory”) can occur in two directions, e.g. for a conrotatory process, both carbons can rotate clockwise or both can rotate counterclockwise. Only one of the two options is shown here. In the previous post we spent a bit more time discussing the two possibilities. The term “torqueselectivity” refers to the propensity of a molecule to favor one of the directions over another, or to use the actual definition: “the preference for inward or outward rotation of substituents in conrotatory or disrotatory electrocyclic reactions”.
Quiz Yourself!
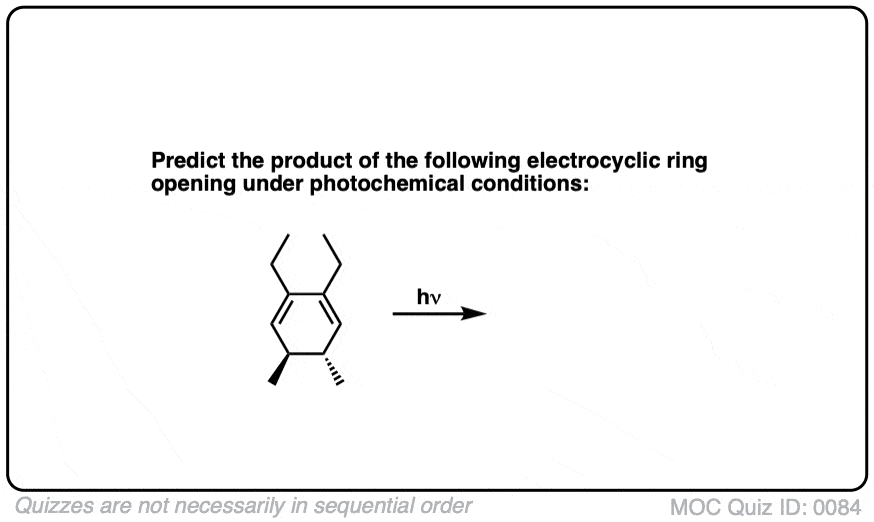
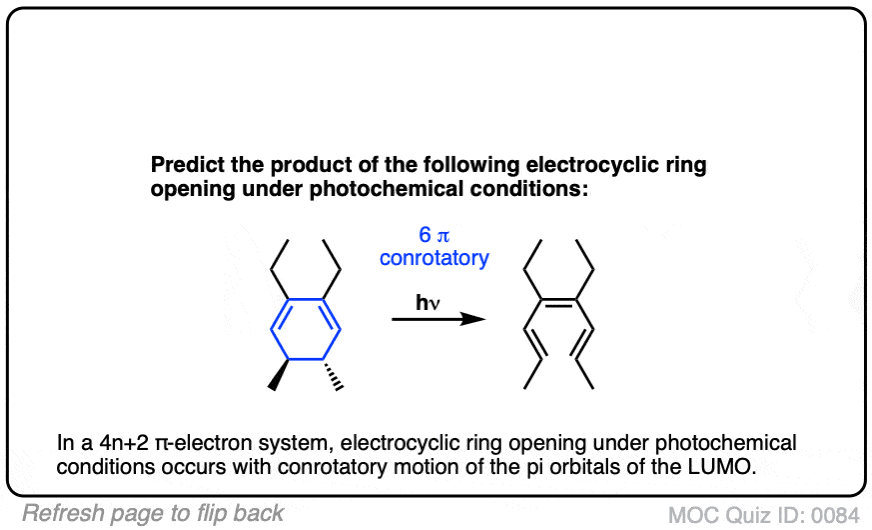
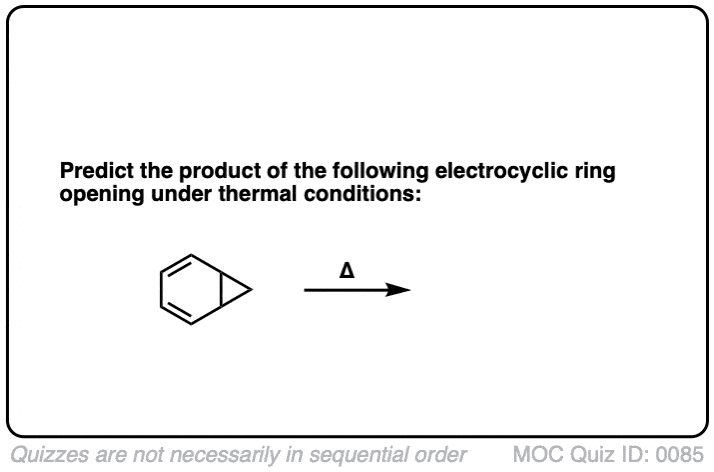
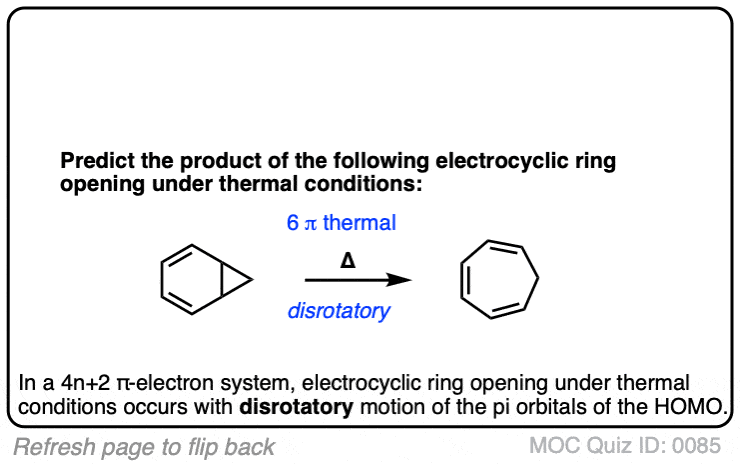
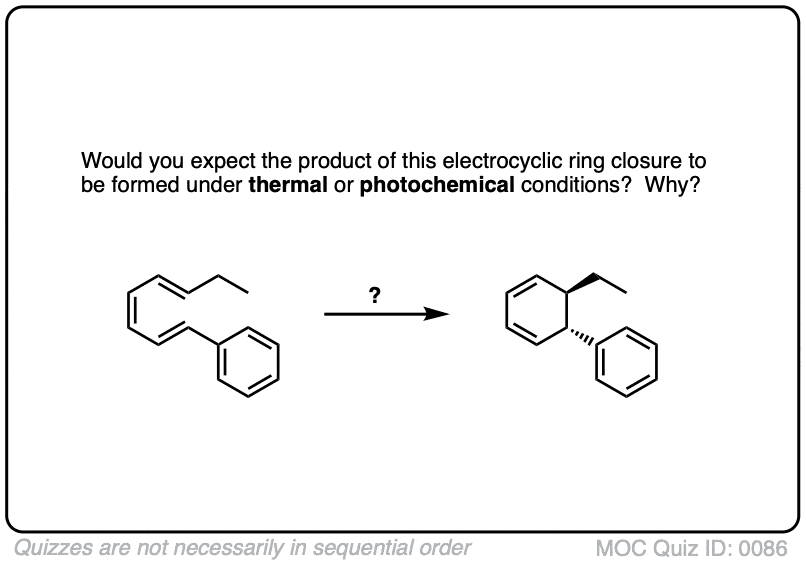
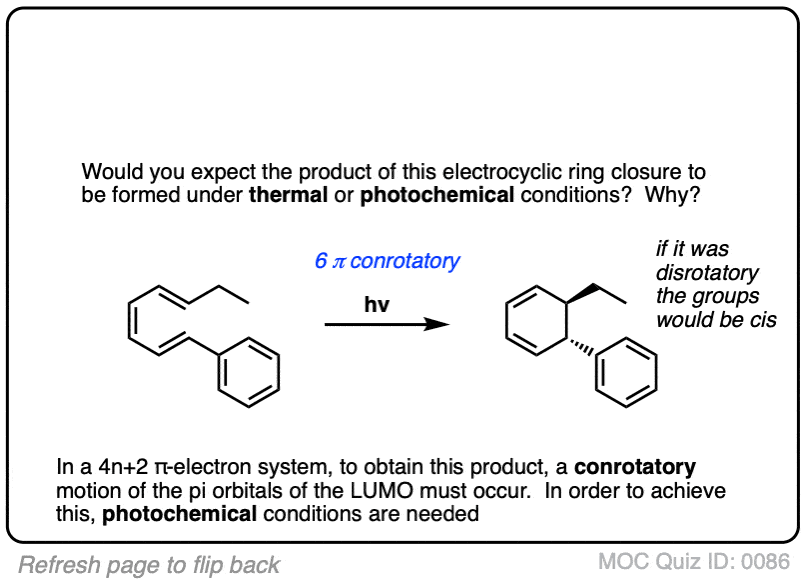
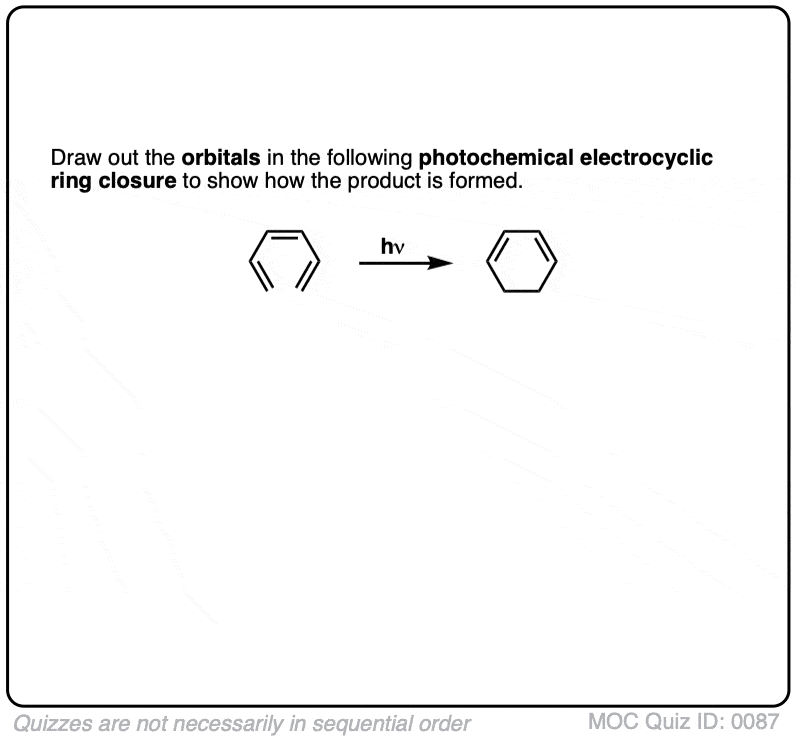
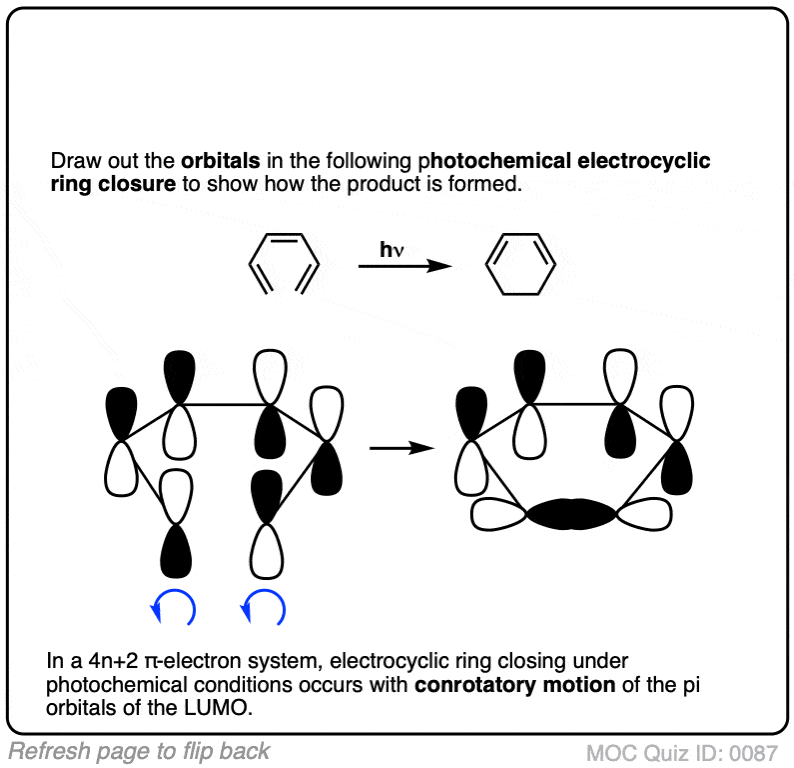
(Advanced) References and Further Reading
These are an extremely common class of reactions, and the prediction of the stereochemistry of these reactions was one of the big successes of Frontier Molecular Orbital (FMO) theory, earning Profs. Roald Hoffmann and Kenichi Fukui the Nobel Prize in Chemistry in 1981. Prof. R. B. Woodward would undoubtedly have shared the prize too (for the development of the eponymous ‘Woodward-Hoffmann’ rules) had he not passed away earlier that year.
- Zur stereospezifität der conrotatorischen cyclisierung des decatetraens
Alexander Dahmen, Rolf Huisgen
Tetrahedron Lett. 1969, 10 (19), 1465-1469
DOI: 10.1016/S0040-4039(01)87915-6 - Stereospecific conrotatory valence isomerization of octatetraenes to cycloocta-1,3,5-trienes
Rolf Huisgen, Alexander Dahmen, and Helmut Huber
Journal of the American Chemical Society 1967, 89 (26), 7130-7131
DOI: 10.1021/ja01002a060 - Electrocyclic reactions of 1-substituted 1,3,5,7-octatetraenes. An ab initio molecular orbital study of torquoselectivity in eight-electron electrocyclizations
Bert E. Thomas, J. D. Evanseck, and K. N. Houk
Journal of the American Chemical Society 1993, 115 (10), 4165-4169
DOI: 10.1021/ja00063a039 - Thermal valence isomerizations: stereochemistry of the 2,4,6-octatriene to 5,6-dimethyl-1,3-cyclohexadiene ring closure
Elliot N. Marvell, Gerald Caple, Bruce Schatz
Tetrahedron Lett. 1965, 6 (7), 385-389
DOI: 10.1016/S0040-4039(00)89964-5 - Ein beitrag zur stereochemie der valenzisomerisierung von trienen mit zentraler cis-doppelbindung zu cyclohexa-1.3-dienen
Vogel, W. Grimme, E. Dinné
Tet. Lett. 1965, 6 (7), 391-395
DOI: 10.1016/S0040-4039(00)89965-7 - Kinetics of thermal interconversion between cis,cis-1,3,5-octatriene, cis,cis,cis-2,4,6-octatriene, and cis,cis,trans-2,4,6-octatriene
John E. Baldwin and V. Prakash Reddy
The Journal of Organic Chemistry 1988, 53 (6), 1129-1132
DOI: 10.1021/jo00241a001 - Theory of stereoselection in conrotatory electrocyclic reactions of substituted cyclobutenes
Nelson G. Rondan and K. N. Houk
Journal of the American Chemical Society 1985, 107 (7), 2099-2111
DOI: 10.1021/ja00293a046 - Conrotatory and disrotatory stationary points for the electrocyclic isomerization of cyclobutene to cis-butadiene
Jacques Breulet and Henry F. Schaefer
Journal of the American Chemical Society 1984, 106 (5), 1221-1226
DOI: 10.1021/ja00317a009 - The endiandric acid cascade. Electrocyclizations in organic synthesis. I. Stepwise, stereocontrolled total synthesis of endiandric acids A and B
K. C. Nicolaou, N. A. Petasis, R. E. Zipkin, and J. Uenishi
Journal of the American Chemical Society 1982, 104 (20), 5555-5557
DOI: 10.1021/ja00384a077 - The endiandric acid cascade. Electrocyclizations in organic synthesis. 2. Stepwise, stereocontrolled total synthesis of endiandric acids C-G
K. C. Nicolaou, N. A. Petasis, J. Uenishi, and R. E. Zipkin
Journal of the American Chemical Society 1982, 104 (20), 5557-5558
DOI: 10.1021/ja00384a078 - The endiandric acid cascade. Electrocyclizations in organic synthesis. 3. “Biomimetic” approach to endiandric acids A-G. Synthesis of precursors
K. C. Nicolaou, R. E. Zipkin, and N. A. Petasis
Journal of the American Chemical Society 1982, 104 (20), 5558-5560
DOI: 10.1021/ja00384a079 - The endiandric acid cascade. Electrocyclizations in organic synthesis. 4. Biomimetic approach to endiandric acids A-G. Total synthesis and thermal studies
K. C. Nicolaou, N. A. Petasis, and R. E. Zipkin
Journal of the American Chemical Society 1982, 104 (20), 5560-5562
DOI: 10.1021/ja00384a080
A series of 4 back-to-back papers in JACS on the synthesis of endiandric acids by Prof. Nicolaou (now at Rice University). Prof. Petasis, a graduate student at the time, was coauthor on all 4 papers, and he is now a professor at USC (Los Angeles, CA). Prof. Petasis is one of the few (or only!) people to have a reaction (Petasis reaction), reagent (Petasis reagent), and rearrangement (Petasis-Ferrier rearrangement) named after him. - Biosynthetic and Biomimetic Electrocyclizations
Christopher M. Beaudry, Jeremiah P. Malerich, and Dirk Trauner
Chemical Reviews 2005, 105 (12), 4757-4778
DOI: 10.1021/cr0406110
Vitamin D biosynthesis is briefly covered here. The ring-opening of dehydrocholesterol is a 6p conroratory electrocyclization. Reactions that would be forbidden thermally are allowed photochemically because different orbitals are involved once an electron is excited. - Conservation of orbital symmetry
Roald Hoffmann and Robert B. Woodward
Accounts of Chemical Research 1968, 1 (1), 17-22
DOI: 1021/ar50001a003
This is an extremely important paper. Conservation of orbital symmetry allows one to predict the stereochemistry of electrocyclic reactions. This paper is a great introduction to the topic by its creators and introduces relevant terms (suprafacial, antarafacial, synrotatory, conrotatory, etc.). Prof. Hoffmann received the Nobel Prize in Chemistry in 1981 for this work, and would undoubtedly have shared it with Prof. Woodward that year (which would have been Woodward’s second Nobel) if he had not passed away untimely the previous year.
C9 and C10 of 7-dehydrocholesterol are likely partially sp2-hybridized due to forced planarity of the sterol backbone, making it different from a generic 4pi => 6pi electrocyclic ring opening. How does this affect the HOMO calculations for the diene prior to electrocyclic ring opening? What is the relationship between the HOMO of the diene reactant (7-dehydrocholesterol) vs. the initially occupied C9-C10 sigma bonding orbital? Is there significant HOMO contributions to electron density between C9 and C10 prior to ring opening?
In the generic inverse reaction (ring closure), the old HOMO (of the 6pi system) becomes the new sigma orbital, which presumably drops in energy below the new HOMO (of the 4pi system). Does this hold true for the hypothetical ring closure of pre-vitamin D3 to 7-dehydrocholesterol, which presumably would be thermal and disrotary?
I’m running some calculations in ORCA, but wow, ORCA is really picky about syntax,