Home / The Williamson Ether Synthesis
Alcohols, Epoxides and Ethers
The Williamson Ether Synthesis
Last updated: February 16th, 2025 |
The Williamson Ether Synthesis
- In the Williamson Ether Synthesis, an alkyl halide (or sulfonate, such as a tosylate or mesylate) undergoes nucleophilic substitution (SN2) by an alkoxide to give an ether.
- Being an SN2 reaction, best results are obtained with primary alkyl halides or methyl halides. Tertiary alkyl halides give elimination instead of ethers. Secondary alkyl halides will give a mixture of elimination and substitution.
- The alkoxide RO– can be those of methyl, primary, secondary, or tertiary alcohols.
- The reaction is often run with a mixture of the alkoxide and its parent alcohol (e.g. NaOEt/EtOH or CH3ONa/CH3OH). Alternatively, a strong base may be added to the alcohol to give the alkoxide. Sodium hydride (NaH) or potassium hydride (KH) are popular choices.
- When an alkoxide and alkyl halide are present on the same molecule, an intramolecular reaction may result to give a new ring. This works best for 5- and 6-membered rings.
- When planning the synthesis of ethers using the Williamson, take care to select the best starting materials for an SN2 reaction. Avoid planning a Williamson involving a tertiary alkyl halide or a phenyl halide!
Table of Contents
-
- The Williamson Ether Synthesis
- Mechanism of the Williamson Ether Synthesis is SN2
- Primary and Methyl Alkyl Halides Work Best
- Solvent Choice In The Williamson
- Intramolecular Williamson Ether Syntheses
- Planning Ether Synthesis via the Williamson
- Summary
- Notes
- Quiz Yourself!
- (Advanced) References and Further Reading
1. The Williamson Ether Synthesis
One of the simplest and most versatile ways for making ethers is the SN2 reaction between an alkoxide (RO–, the conjugate base of an alcohol) and an alkyl halide.
Although this is a very old reaction – the first report was in 1850! – it just hasn’t been surpassed. It works well for making a variety of ethers and is known as the Williamson Ether Synthesis.
SN2 reactions between neutral alcohols and alkyl halides are generally quite slow. Since the conjugate base of any species is a better nucleophile, the reaction is sped up considerably (Note 1) by employing an alkoxide instead of a neutral alcohol. [See article: What Makes A Good Nucleophile?]
A common way to do the Williamson is to simply use the alkoxide nucleophile with its parent alcohol as solvent (indeed, that’s how it was done in 1850!)
For example, the classic way to make diethyl ether is to treat the ethyl halide (the chloride, bromide, or iodide all work, but not the fluoride) with the ethoxide ion in ethanol.
For our purposes the identity of the leaving group (iodide, bromide, chloride, tosylate (OTs), mesylate (OMs) ) does not really matter, although it is important to know their relative leaving group abilities (See article: What Makes A Good Leaving Group).
Likewise the identity of the alkali metal salt (Li+ , Na+, K+, etc.) does not matter much for our purposes and we will use these metal salts interchangeably.
Since it’s not always an option to use the alcohol as solvent, another option is to generate the alkoxide by using a strong base that will irreversibly deprotonate the alcohol.
A popular choice is the hydride ion H– , which is the conjugate base of hydrogen gas (pKa about 35) and is also a poor nucleophile to boot. Sodium hydride (NaH) or potassium hydride (KH) can be added to the starting alcohol to generate the alkoxide. The hydrogen gas byproduct then bubbles out of solution into the atmosphere.
2. The Williamson Ether Synthesis Proceeds Through an SN2 Mechanism
The Williamson ether synthesis is a substitution reaction, where a bond is formed and broken on the same carbon atom. In this substitution reaction, a new C-O bond is formed, and a bond is broken between the carbon and the leaving group (LG) which is typically a halide or sulfonate.
It proceeds through an SN2 mechanism (nucleophilic substitution, bimolecular) where the nucleophile approaches the carbon atom from the backside of the carbon-leaving group bond. (See article: The SN2 Mechanism)
A pair of electrons from the nucleophile are donated into the sigma* (antibonding) orbital of the C-leaving group bond.
This requires that the nucleophile actually makes its way to the orbital on the backside of the carbon! For this reason the SN2 is fastest for methyl and primary alkyl halides, and does not occur on tertiary alkyl halides due to the fact that nucleophiles can’t make their way through the tangled thicket of alkyl groups on the backside. (See article: Steric Hindrance Is Like A Fat Goalie) .
Substitution reactions of alkoxides with secondary alkyl halides can occur, but often occur with significant elimination through the E2 pathway.
In the transition state of the SN2 there is a five-coordinate geometry about the carbon with partial bonds to the nucleophile and to the leaving group.
As the carbon-nucleophile bond strengthens and the carbon-leaving group bond weakens, the geometry of the carbon becomes inverted, like the proverbial umbrella in a strong wind. This is generally not noticeable unless the alkyl halide carbon is a chiral center.
3. Primary and Methyl Alkyl Halides Work Best
The Williamson works best for primary and methyl alkyl halides. Let’s look at some examples:
Note that the third example the stereochemistry of the C-O bond is unaffected. That’s because it’s only the geometry of the electrophile (i.e. the alkyl halide) in the SN2 that becomes inverted.
If the alkyl halide carbon is chiral, inversion can occur, as it does for this secondary alkyl halide below.
Since alkoxides are strong bases, there will be significant competition between SN2 and E2 reactions with secondary alkyl halides. Alkenes and ethers are generally obtained as mixtures. [Note 2].
With tertiary alkyl halides, the Williamson ether reaction fails completely, and only alkenes are obtained.
Since SN2 and E2 reactions generally do not occur with sp2 hybridized carbons, another case where Williamson reactions fail is with aryl and alkenyl halides.
4. Choice of Solvent For Ether Formation
A common choice of solvent for the Williamson is to use the parent alcohol of the alkoxide, such as ethanol when using sodium ethoxide.
It is generally a bad idea to use an alcoholic solvent that is not the conjugate acid of the alkoxide, as discussed in the quiz below:
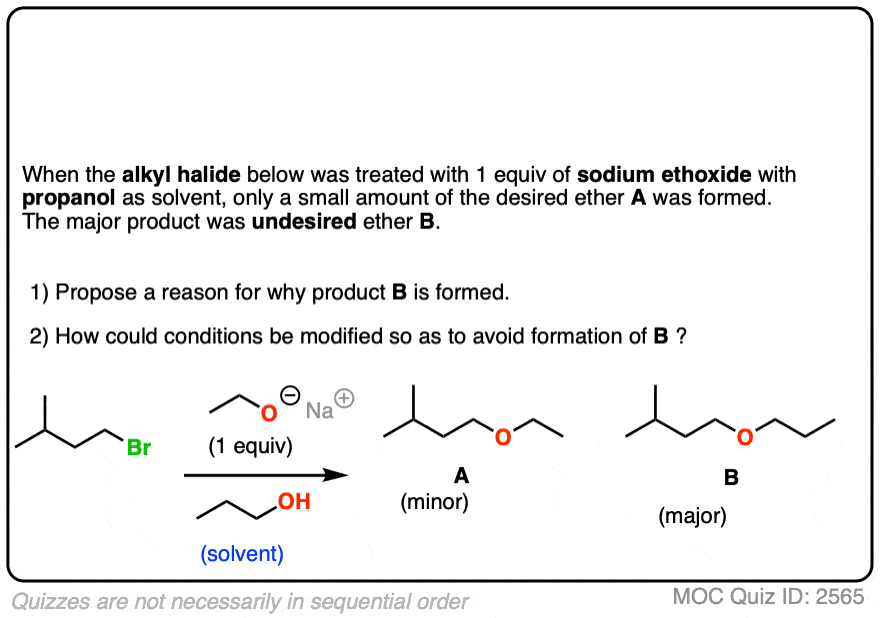
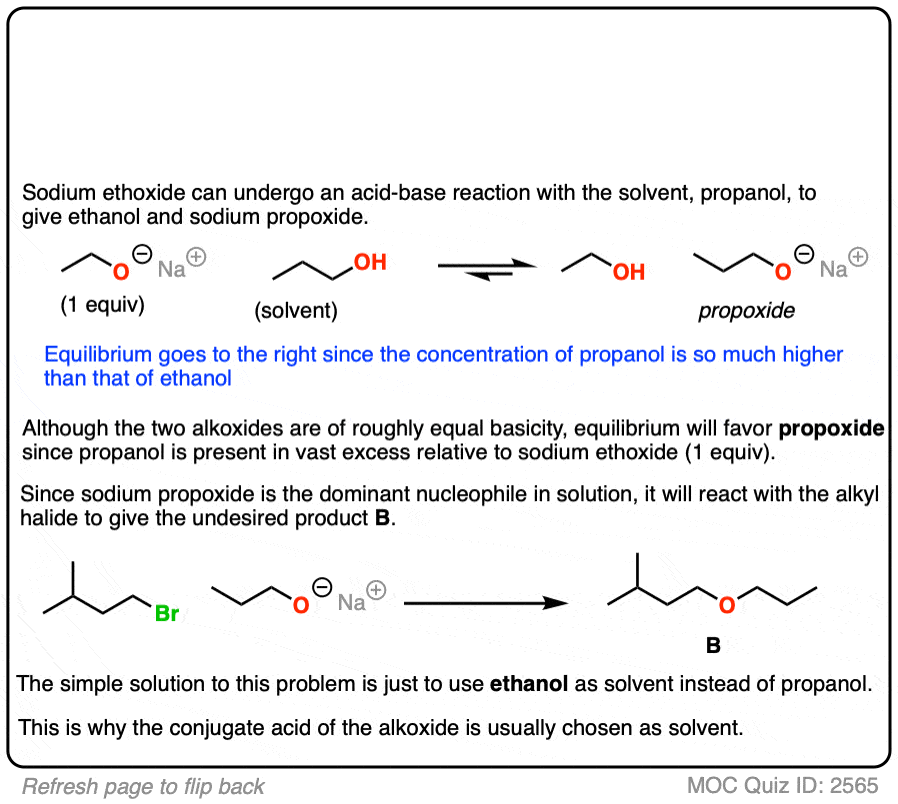
When using sodium hydride (NaH) or potassium hydride (KH) a common choice of solvent is ethers such as tetrahydrofuran (THF), diethyl ether, or polar aprotic solvents such as dimethylsulfoxide (DMSO).
5. Intramolecular Williamson Reactions
If an alcohol and alkyl halide are present on the same molecule, there is the potential for an intramolecular Williamson ether reaction to occur.
This will result in a new ring.
Ring formation is best for 5- and 6-membered rings. [Note 3 ]
The mechanism for the intramolecular Williamson reaction is identical to that for a normal SN2; it just looks weird the first time you see it.
See if you can draw the mechanism:
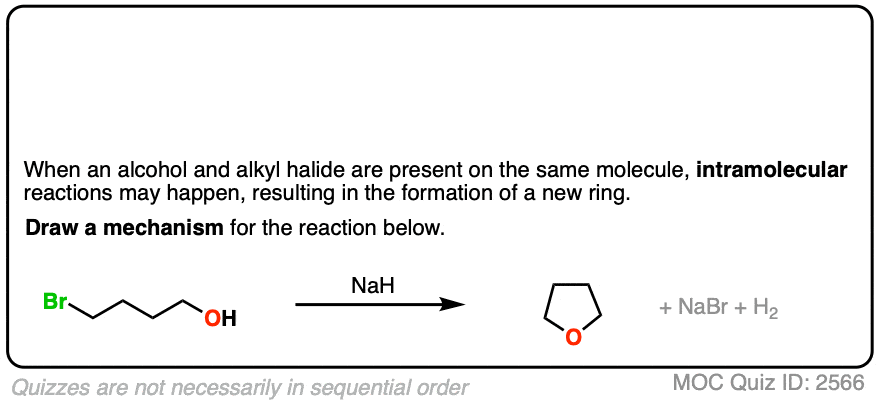
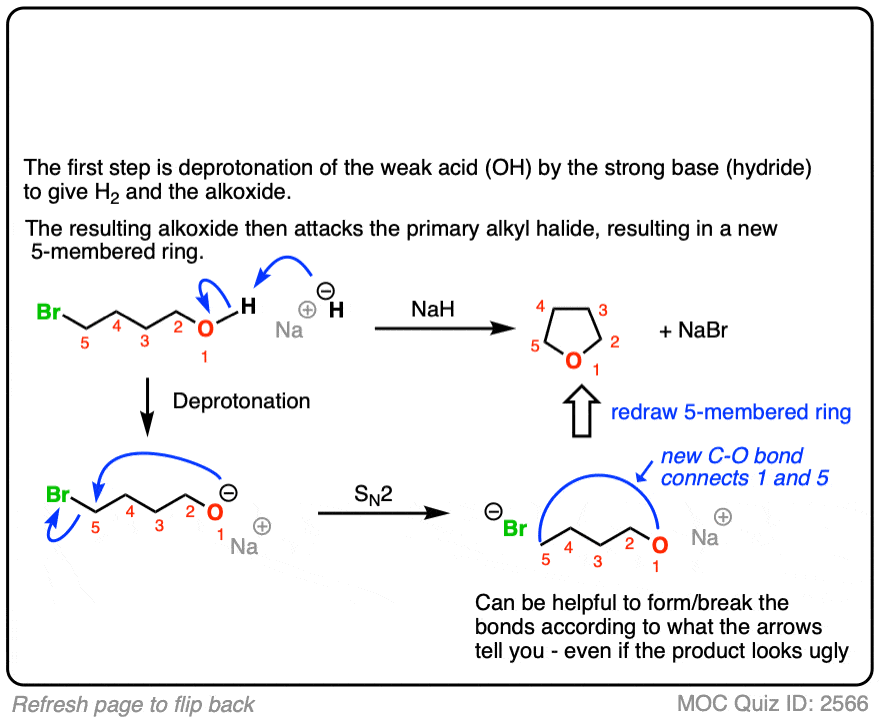
It’s also valuable to be able to work backwards from a final product to propose plausible starting materials. See if you can draw a feasible starting material that will result in the Williamson product below:
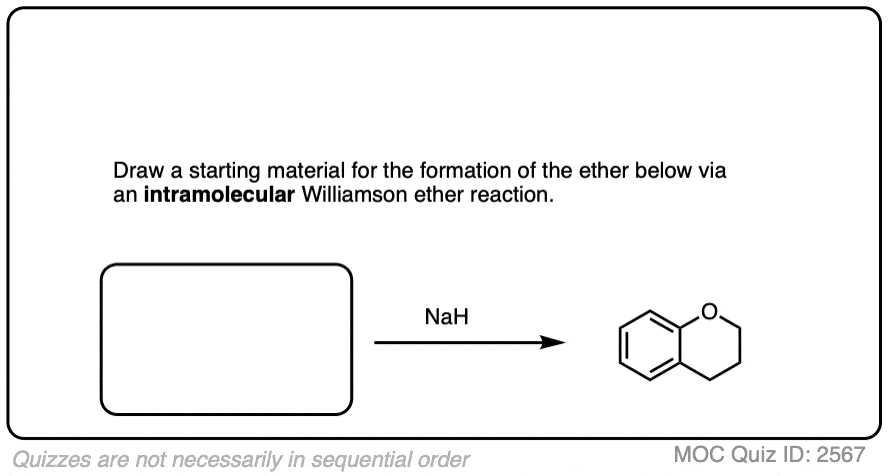
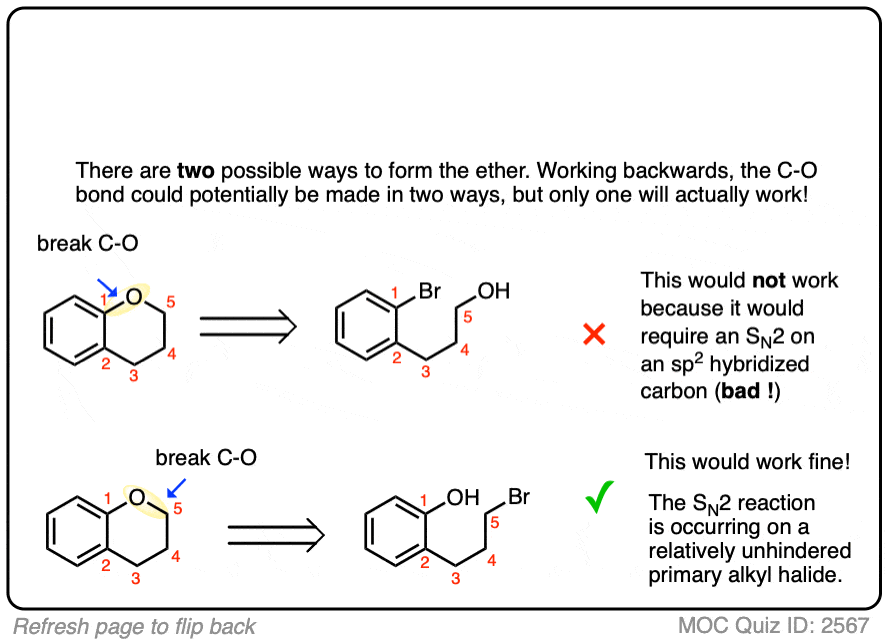
6. Planning Syntheses of Ethers via The Williamson
All right. Given all we have said about the Williamson, let’s see some examples of using this reaction to plan some syntheses of ethers.
With any ether there are two potential sites where a new C-O bond could be formed, which gives you two alkyl halide + alkoxide combinations to choose from.
Let’s start with a fairly easy example. See if you can come up with reasonable starting materials for the synthesis of propyl methyl ether via the Williamson reaction.
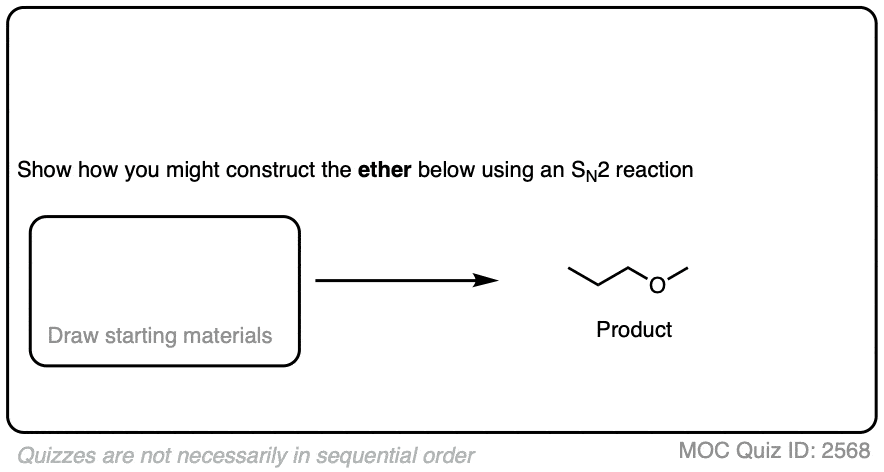
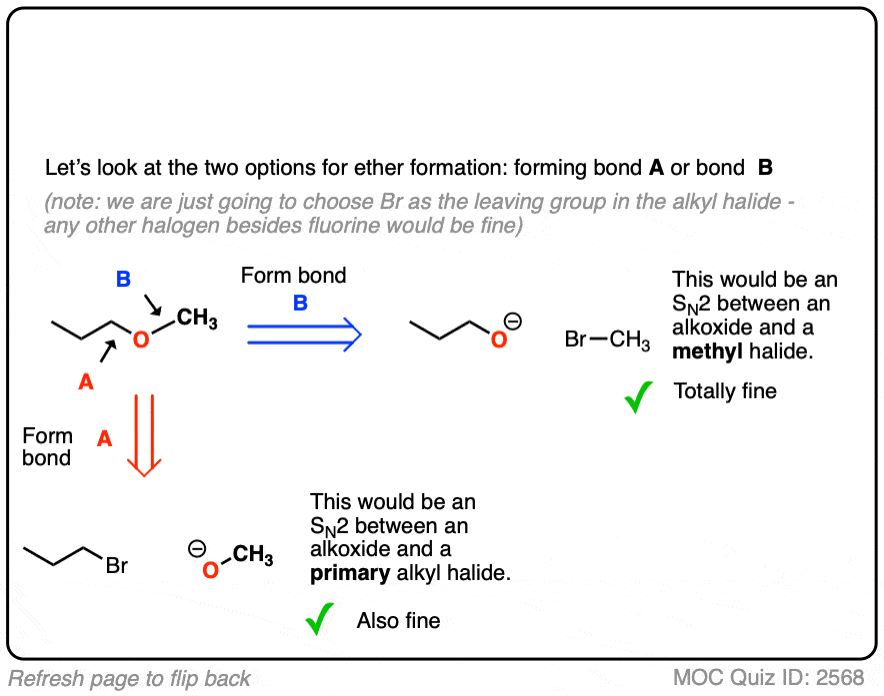
Note that for our purposes it doesn’t matter whether you choose Cl, Br, I or another good leaving group for your alkyl halide/sulfonate, so long that it isn’t F.
With propyl methyl ether, there are actually two good choices for building the ether. You can either use propyl halide (primary) with methoxide, or methyl halide (methyl) with propoxide. Both of these SN2 reactions should work well.
Since elimination is absolutely impossible on methyl, I’d give a slight preference to using the propoxide/CH3Br combination, but either one would work.
Ready for the next one? See if you can come up with a plan for the ether below:
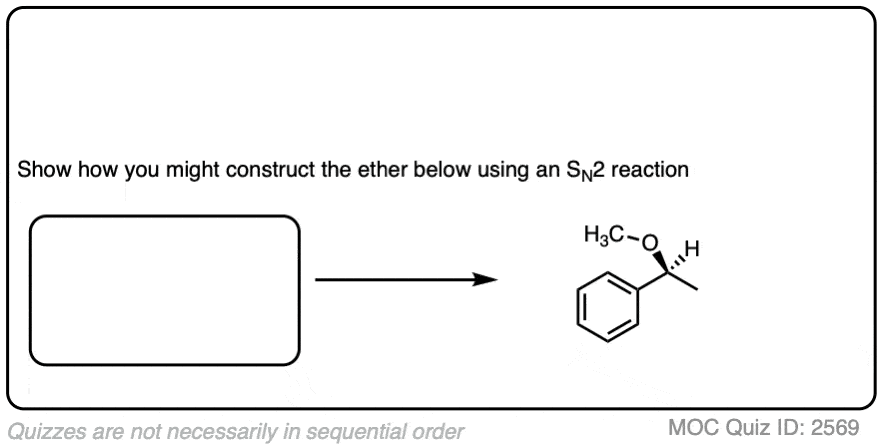
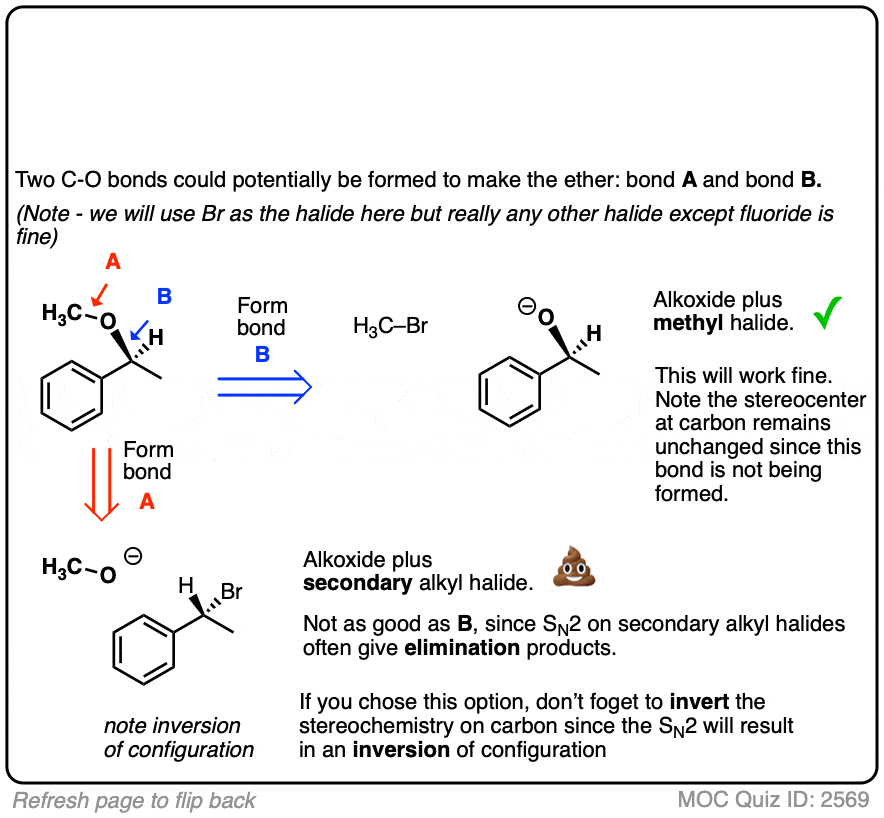
Working backwards gives us two sets of reactants. The one that will work best will be the alkoxide of the secondary alcohol with a methyl halide, since SN2 reactions are fastest on methyl groups and elimination reactions (E2) are impossible and will not compete.
A worse choice would be a secondary alkyl halide with methoxide. Note that since this is an SN2 – which proceeds with inversion of configuration – and the secondary alkyl halide chiral, the starting alkyl halide will have the opposite configuration to that of the starting material.
Let’s try t-butyl ethyl ether next.
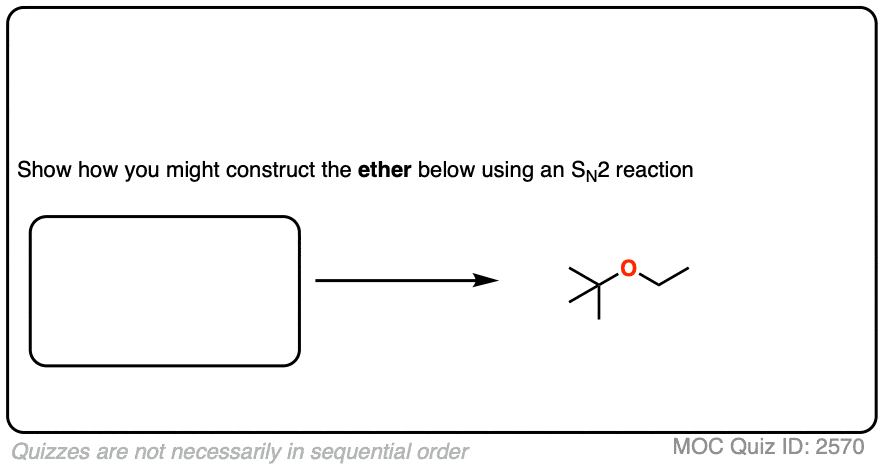
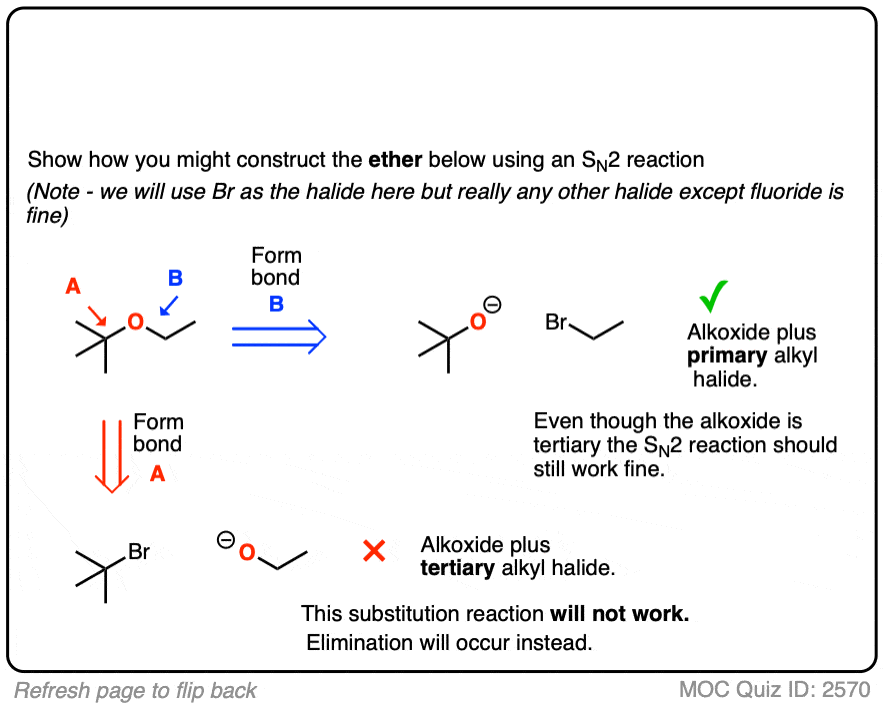
Our two choices of reactants include
- A tertiary alkoxide with a primary alkyl halide
- A tertiary alkyl halide with a primary alkoxide
Hopefully by now it should be clear that the first choice is best, since primary alkyl halides are excellent substrates for SN2 reactions and tertiary alkyl halides are not.
Even though the alkoxide is tertiary, the reaction should still work fairly well. Being bulky, there will be more elimination (E2) than normal, but this could be minimized by keeping the reaction temperature relatively low. [See article: Bulky Bases In Elimination Reactions]
Our final quiz asks how to synthesize phenyl methyl ether.
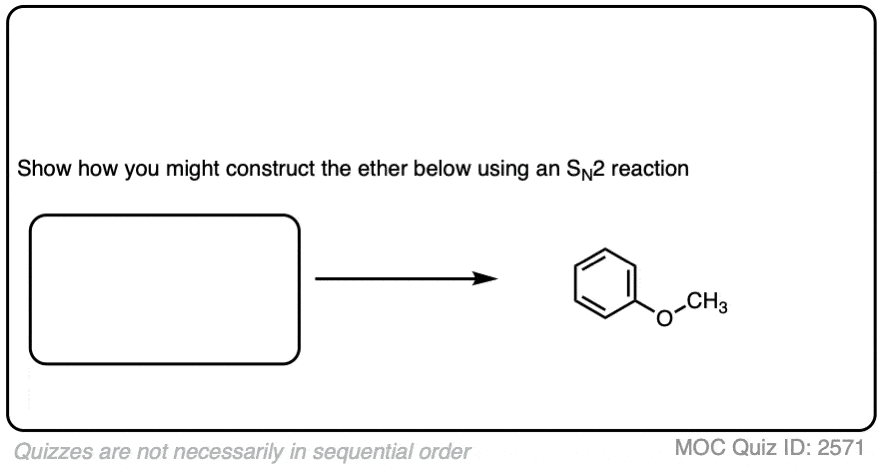
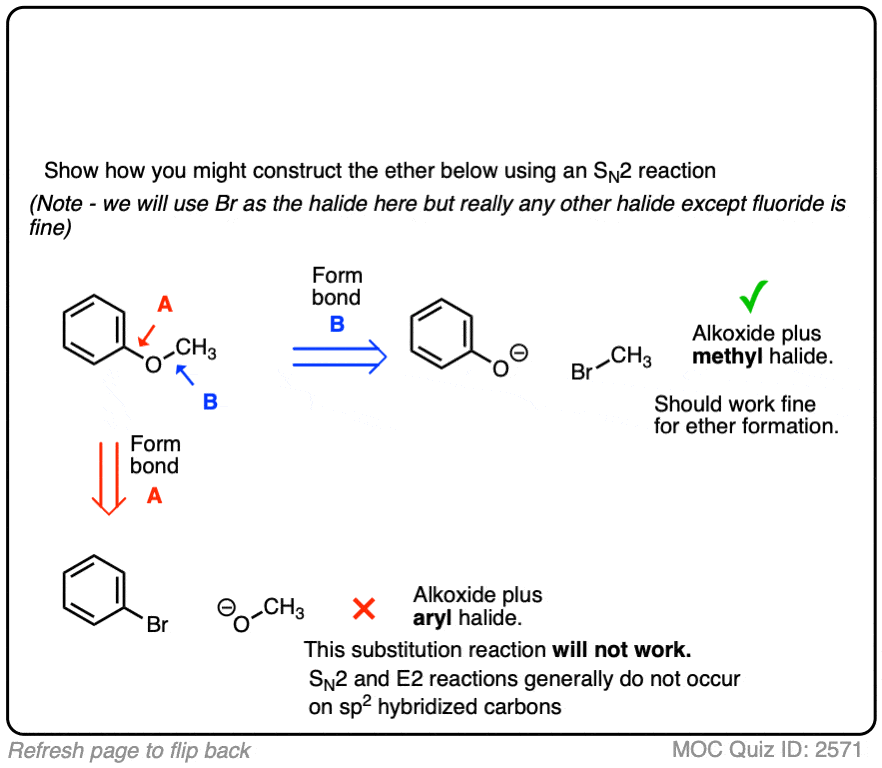
Our choices are:
- A methyl halide with a phenyl alkoxide
- An aryl halide with methoxide
Again, there is a very clear good choice here, as sp2 hybridized carbons (aryl halides) do not undergo SN2 reactions (just try doing a backside attack inside that phenyl ring!). In constrast, the reaction between the phenoxide and CH3Br should work much better without any complication.
7. Summary
So what are the key takeaways here?
If you’ve already learned the SN2 reaction, this should mainly be a refresher.
- SN2 reactions work well for methyl and primary alkyl halides, don’t work for tertiary, alkenyl or aryl halides, and are pretty borderline for secondary alkyl halides.
- Make sure you are familiar with drawing intramolecular examples of this reaction, because instructors tend to love throwing these types of mechanisms at you on exams.
- Get comfortable with planning the synthesis of ethers through Williamson reactions. There will generally be two alkoxide/alkyl halide combinations to choose from. Choose the best SN2 reaction available.
There are situations where the Williamson is not the best choice for ether synthesis and we must resort to other methods. For more, see Ethers From Alkenes, Tertiary Alkyl Halides, and Oxymercuration.
Notes
Related Articles
- Ethers From Alkenes, Tertiary Alkyl Halides and Alkoxymercuration
- Steric Hindrance is Like a Fat Goalie
- What Makes A Good Nucleophile?
- What makes a good leaving group?
- The SN2 Mechanism
- The E2 Mechanism
- Alcohols Can Act As Acids Or Bases (And Why It Matters)
- Bulky Bases in Elimination Reactions
- Intramolecular Reactions of Alcohols and Ethers
The contents of a separate article, “The Williamson Ether Synthesis – Planning” has been combined into this article – Sept 2023.
Note 1 – [Background rate of SN2]
Note 2 – [Secondary alkyl halide]
Note 3 – A Final Note About Rates of Ring Formation
I should end with a cautionary note about reactions that lead to ring formation. One question that comes up a lot is, “when do I know when a new ring will form?” [Shortcut spoiler: yes to 5 and 6 (and 3), generally “no” to rings 7 and above]
Great question! This is one of those issues that makes organic chemistry “hard” for the beginner, but “deep” and “interesting” for the lifelong practitioner because there are several key factors that often work in opposite directions.
First of all: Not all rings form at the same rate. That is, the rate at which a ring will form is, to some extent, dependent on the length of the chain.
How does this fit in with what we already know about substitution reactions?
Remember that the rate of a substitution reaction is proportional to the concentration of nucleophile and the concentration of electrophile. But what happens when the nucleophile and electrophile are on the same molecule? For this we use a concept called “effective concentration” which is to say that the reaction rate will be related to how much time the nucleophile spends in the vicinity of the electrophile. There isn’t space to go into this in detail in this post, but let’s use this velcro straps on this shoe as an overly simplistic example.
If the velcro straps are too short, then the nucleophile (strap) can’t reach the electrophile (on the shoe). If the velcro straps are too long (imagine if they were each a foot long, for instance!) , then the shoes will be annoying to put on because of the decreasing likelihood that the nucleophile will be in the vicinity of the electrophile (an example of low “effective concentration”). The rate of formation for very large rings will approach the rate of intermolecular reactions.
Of course molecules are more complicated than belts (or velcro straps) because of the ideal 109° angles of tetrahedral carbons. That creates some additional complexity, notably the issue of ring strain.
For example you are probably aware by this point that 3 and 4 membered rings are quite strained, whereas rings of size 5, 6, and 7 are relatively unstrained. [See article – Ring Strain in Cyclopropane and Cyclobutane]
If you’re just starting out you’re likely unaware that rings of size 8-11 are strained for a very interesting reason (transannular strain) and then rings of size 12 and above are generally unstrained.
For a student in an introductory course, a good rule of thumb is: Formation of 5 and 6 membered rings is fast. Formation of rings of size 7 and above is slow. As for the smaller ring sizes, we’ve seen examples where 3 membered rings form (from halohydrins). Seen less often, but also fast is the formation of 4 membered rings.
This is a vague generalization. “Give me numbers!” you might be saying. My copy of March says the following. Note that this is for a different reaction than the Williamson ether synthesis [formation of cyclic esters through SN2 of carboxylates with alkyl halides], but the trend should hold.
For a more quantitative approach, I suggest you look into this paper on ring closure kinetics.
Quiz Yourself!
Become a MOC member to see the clickable quiz with answers on the back.
Become a MOC member to see the clickable quiz with answers on the back.
Become a MOC member to see the clickable quiz with answers on the back.
Become a MOC member to see the clickable quiz with answers on the back.
Become a MOC member to see the clickable quiz with answers on the back.
Become a MOC member to see the clickable quiz with answers on the back.
(Advanced) References and Further Reading
- XLV. Theory of ætherification.
Alexander Williamson (1850) , The London, Edinburgh, and Dublin Philosophical Magazine and Journal of Science, 37:251, 350-356,
DOI: 080/14786445008646627
The original Williamson paper. When Williamson reported the reaction in 1850 he didn’t know what an SN2 was – scientists didn’t even know what electrons were, for that matter – which again goes to show that the science of organic chemistry developed through a lot of empirical observations first, and the theory developed later. - Equilenin 3-Benzyl Ether
M. Hoehn, Clifford R. Dorn, and Bernard A. Nelson
The Journal of Organic Chemistry 1965 30 (1), 316-316
DOI: DOI: 10.1021/jo01012a520
One of the reactions in this paper is a classic Williamson reaction – protection of the alcohol in dehydroestrone as a benzyl ether, using benzyl chloride. - Total Synthesis of (+)-7-Deoxypancratistatin: A Radical Cyclization Approach
Gary E. Keck, Stanton F. McHardy, and Jerry A. Murry
Journal of the American Chemical Society 1995 117 (27), 7289-7290
DOI: 1021/ja00132a047
In modern organic synthesis, the Williamson reaction is used for the protection of reactive alcohols in a substrate. Common protecting groups include methoxymethyl (MOM) and 2-methoxyethoxymethyl (MEM). MOM protection is employed in this total synthesis by Prof. Keck and coworkers.
00 General Chemistry Review
01 Bonding, Structure, and Resonance
- How Do We Know Methane (CH4) Is Tetrahedral?
- Hybrid Orbitals and Hybridization
- How To Determine Hybridization: A Shortcut
- Orbital Hybridization And Bond Strengths
- Sigma bonds come in six varieties: Pi bonds come in one
- A Key Skill: How to Calculate Formal Charge
- The Four Intermolecular Forces and How They Affect Boiling Points
- 3 Trends That Affect Boiling Points
- How To Use Electronegativity To Determine Electron Density (and why NOT to trust formal charge)
- Introduction to Resonance
- How To Use Curved Arrows To Interchange Resonance Forms
- Evaluating Resonance Forms (1) - The Rule of Least Charges
- How To Find The Best Resonance Structure By Applying Electronegativity
- Evaluating Resonance Structures With Negative Charges
- Evaluating Resonance Structures With Positive Charge
- Exploring Resonance: Pi-Donation
- Exploring Resonance: Pi-acceptors
- In Summary: Evaluating Resonance Structures
- Drawing Resonance Structures: 3 Common Mistakes To Avoid
- How to apply electronegativity and resonance to understand reactivity
- Bond Hybridization Practice
- Structure and Bonding Practice Quizzes
- Resonance Structures Practice
02 Acid Base Reactions
- Introduction to Acid-Base Reactions
- Acid Base Reactions In Organic Chemistry
- The Stronger The Acid, The Weaker The Conjugate Base
- Walkthrough of Acid-Base Reactions (3) - Acidity Trends
- Five Key Factors That Influence Acidity
- Acid-Base Reactions: Introducing Ka and pKa
- How to Use a pKa Table
- The pKa Table Is Your Friend
- A Handy Rule of Thumb for Acid-Base Reactions
- Acid Base Reactions Are Fast
- pKa Values Span 60 Orders Of Magnitude
- How Protonation and Deprotonation Affect Reactivity
- Acid Base Practice Problems
03 Alkanes and Nomenclature
- Meet the (Most Important) Functional Groups
- Condensed Formulas: Deciphering What the Brackets Mean
- Hidden Hydrogens, Hidden Lone Pairs, Hidden Counterions
- Don't Be Futyl, Learn The Butyls
- Primary, Secondary, Tertiary, Quaternary In Organic Chemistry
- Branching, and Its Affect On Melting and Boiling Points
- The Many, Many Ways of Drawing Butane
- Wedge And Dash Convention For Tetrahedral Carbon
- Common Mistakes in Organic Chemistry: Pentavalent Carbon
- Table of Functional Group Priorities for Nomenclature
- Summary Sheet - Alkane Nomenclature
- Organic Chemistry IUPAC Nomenclature Demystified With A Simple Puzzle Piece Approach
- Boiling Point Quizzes
- Organic Chemistry Nomenclature Quizzes
04 Conformations and Cycloalkanes
- Staggered vs Eclipsed Conformations of Ethane
- Conformational Isomers of Propane
- Newman Projection of Butane (and Gauche Conformation)
- Introduction to Cycloalkanes
- Geometric Isomers In Small Rings: Cis And Trans Cycloalkanes
- Calculation of Ring Strain In Cycloalkanes
- Cycloalkanes - Ring Strain In Cyclopropane And Cyclobutane
- Cyclohexane Conformations
- Cyclohexane Chair Conformation: An Aerial Tour
- How To Draw The Cyclohexane Chair Conformation
- The Cyclohexane Chair Flip
- The Cyclohexane Chair Flip - Energy Diagram
- Substituted Cyclohexanes - Axial vs Equatorial
- Ranking The Bulkiness Of Substituents On Cyclohexanes: "A-Values"
- Cyclohexane Chair Conformation Stability: Which One Is Lower Energy?
- Fused Rings - Cis-Decalin and Trans-Decalin
- Naming Bicyclic Compounds - Fused, Bridged, and Spiro
- Bredt's Rule (And Summary of Cycloalkanes)
- Newman Projection Practice
- Cycloalkanes Practice Problems
05 A Primer On Organic Reactions
- The Most Important Question To Ask When Learning a New Reaction
- Curved Arrows (for reactions)
- Nucleophiles and Electrophiles
- The Three Classes of Nucleophiles
- Nucleophilicity vs. Basicity
- What Makes A Good Nucleophile?
- What Makes A Good Leaving Group?
- 3 Factors That Stabilize Carbocations
- Equilibrium and Energy Relationships
- 7 Factors that stabilize negative charge in organic chemistry
- 7 Factors That Stabilize Positive Charge in Organic Chemistry
- What's a Transition State?
- Hammond's Postulate
- Learning Organic Chemistry Reactions: A Checklist (PDF)
- Introduction to Oxidative Cleavage Reactions
06 Free Radical Reactions
- Free Radical Reactions
- 3 Factors That Stabilize Free Radicals
- Bond Strengths And Radical Stability
- Free Radical Initiation: Why Is "Light" Or "Heat" Required?
- Initiation, Propagation, Termination
- Monochlorination Products Of Propane, Pentane, And Other Alkanes
- Selectivity In Free Radical Reactions
- Selectivity in Free Radical Reactions: Bromination vs. Chlorination
- Halogenation At Tiffany's
- Allylic Bromination
- Bonus Topic: Allylic Rearrangements
- In Summary: Free Radicals
- Synthesis (2) - Reactions of Alkanes
- Free Radicals Practice Quizzes
07 Stereochemistry and Chirality
- Types of Isomers: Constitutional Isomers, Stereoisomers, Enantiomers, and Diastereomers
- How To Draw The Enantiomer Of A Chiral Molecule
- How To Draw A Bond Rotation
- Introduction to Assigning (R) and (S): The Cahn-Ingold-Prelog Rules
- Assigning Cahn-Ingold-Prelog (CIP) Priorities (2) - The Method of Dots
- Enantiomers vs Diastereomers vs The Same? Two Methods For Solving Problems
- Assigning R/S To Newman Projections (And Converting Newman To Line Diagrams)
- How To Determine R and S Configurations On A Fischer Projection
- The Meso Trap
- Optical Rotation, Optical Activity, and Specific Rotation
- Optical Purity and Enantiomeric Excess
- What's a Racemic Mixture?
- Chiral Allenes And Chiral Axes
- Stereochemistry Practice Problems and Quizzes
08 Substitution Reactions
- Nucleophilic Substitution Reactions - Introduction
- Two Types of Nucleophilic Substitution Reactions
- The SN2 Mechanism
- Why the SN2 Reaction Is Powerful
- The SN1 Mechanism
- The Conjugate Acid Is A Better Leaving Group
- Comparing the SN1 and SN2 Reactions
- Polar Protic? Polar Aprotic? Nonpolar? All About Solvents
- Steric Hindrance is Like a Fat Goalie
- Common Blind Spot: Intramolecular Reactions
- Substitution Practice - SN1
- Substitution Practice - SN2
09 Elimination Reactions
- Elimination Reactions (1): Introduction And The Key Pattern
- Elimination Reactions (2): The Zaitsev Rule
- Elimination Reactions Are Favored By Heat
- Two Elimination Reaction Patterns
- The E1 Reaction
- The E2 Mechanism
- E1 vs E2: Comparing the E1 and E2 Reactions
- Antiperiplanar Relationships: The E2 Reaction and Cyclohexane Rings
- Bulky Bases in Elimination Reactions
- Comparing the E1 vs SN1 Reactions
- Elimination (E1) Reactions With Rearrangements
- E1cB - Elimination (Unimolecular) Conjugate Base
- Elimination (E1) Practice Problems And Solutions
- Elimination (E2) Practice Problems and Solutions
10 Rearrangements
11 SN1/SN2/E1/E2 Decision
- Identifying Where Substitution and Elimination Reactions Happen
- Deciding SN1/SN2/E1/E2 (1) - The Substrate
- Deciding SN1/SN2/E1/E2 (2) - The Nucleophile/Base
- SN1 vs E1 and SN2 vs E2 : The Temperature
- Deciding SN1/SN2/E1/E2 - The Solvent
- Wrapup: The Key Factors For Determining SN1/SN2/E1/E2
- Alkyl Halide Reaction Map And Summary
- SN1 SN2 E1 E2 Practice Problems
12 Alkene Reactions
- E and Z Notation For Alkenes (+ Cis/Trans)
- Alkene Stability
- Alkene Addition Reactions: "Regioselectivity" and "Stereoselectivity" (Syn/Anti)
- Stereoselective and Stereospecific Reactions
- Hydrohalogenation of Alkenes and Markovnikov's Rule
- Hydration of Alkenes With Aqueous Acid
- Rearrangements in Alkene Addition Reactions
- Halogenation of Alkenes and Halohydrin Formation
- Oxymercuration Demercuration of Alkenes
- Hydroboration Oxidation of Alkenes
- m-CPBA (meta-chloroperoxybenzoic acid)
- OsO4 (Osmium Tetroxide) for Dihydroxylation of Alkenes
- Palladium on Carbon (Pd/C) for Catalytic Hydrogenation of Alkenes
- Cyclopropanation of Alkenes
- A Fourth Alkene Addition Pattern - Free Radical Addition
- Alkene Reactions: Ozonolysis
- Summary: Three Key Families Of Alkene Reaction Mechanisms
- Synthesis (4) - Alkene Reaction Map, Including Alkyl Halide Reactions
- Alkene Reactions Practice Problems
13 Alkyne Reactions
- Acetylides from Alkynes, And Substitution Reactions of Acetylides
- Partial Reduction of Alkynes With Lindlar's Catalyst
- Partial Reduction of Alkynes With Na/NH3 To Obtain Trans Alkenes
- Alkyne Hydroboration With "R2BH"
- Hydration and Oxymercuration of Alkynes
- Hydrohalogenation of Alkynes
- Alkyne Halogenation: Bromination and Chlorination of Alkynes
- Oxidation of Alkynes With O3 and KMnO4
- Alkenes To Alkynes Via Halogenation And Elimination Reactions
- Alkynes Are A Blank Canvas
- Synthesis (5) - Reactions of Alkynes
- Alkyne Reactions Practice Problems With Answers
14 Alcohols, Epoxides and Ethers
- Alcohols - Nomenclature and Properties
- Alcohols Can Act As Acids Or Bases (And Why It Matters)
- Alcohols - Acidity and Basicity
- The Williamson Ether Synthesis
- Ethers From Alkenes, Tertiary Alkyl Halides and Alkoxymercuration
- Alcohols To Ethers via Acid Catalysis
- Cleavage Of Ethers With Acid
- Epoxides - The Outlier Of The Ether Family
- Opening of Epoxides With Acid
- Epoxide Ring Opening With Base
- Making Alkyl Halides From Alcohols
- Tosylates And Mesylates
- PBr3 and SOCl2
- Elimination Reactions of Alcohols
- Elimination of Alcohols To Alkenes With POCl3
- Alcohol Oxidation: "Strong" and "Weak" Oxidants
- Demystifying The Mechanisms of Alcohol Oxidations
- Protecting Groups For Alcohols
- Thiols And Thioethers
- Calculating the oxidation state of a carbon
- Oxidation and Reduction in Organic Chemistry
- Oxidation Ladders
- SOCl2 Mechanism For Alcohols To Alkyl Halides: SN2 versus SNi
- Alcohol Reactions Roadmap (PDF)
- Alcohol Reaction Practice Problems
- Epoxide Reaction Quizzes
- Oxidation and Reduction Practice Quizzes
15 Organometallics
- What's An Organometallic?
- Formation of Grignard and Organolithium Reagents
- Organometallics Are Strong Bases
- Reactions of Grignard Reagents
- Protecting Groups In Grignard Reactions
- Synthesis Problems Involving Grignard Reagents
- Grignard Reactions And Synthesis (2)
- Organocuprates (Gilman Reagents): How They're Made
- Gilman Reagents (Organocuprates): What They're Used For
- The Heck, Suzuki, and Olefin Metathesis Reactions (And Why They Don't Belong In Most Introductory Organic Chemistry Courses)
- Reaction Map: Reactions of Organometallics
- Grignard Practice Problems
16 Spectroscopy
- Degrees of Unsaturation (or IHD, Index of Hydrogen Deficiency)
- Conjugation And Color (+ How Bleach Works)
- Introduction To UV-Vis Spectroscopy
- UV-Vis Spectroscopy: Absorbance of Carbonyls
- UV-Vis Spectroscopy: Practice Questions
- Bond Vibrations, Infrared Spectroscopy, and the "Ball and Spring" Model
- Infrared Spectroscopy: A Quick Primer On Interpreting Spectra
- IR Spectroscopy: 4 Practice Problems
- 1H NMR: How Many Signals?
- Homotopic, Enantiotopic, Diastereotopic
- Diastereotopic Protons in 1H NMR Spectroscopy: Examples
- 13-C NMR - How Many Signals
- Liquid Gold: Pheromones In Doe Urine
- Natural Product Isolation (1) - Extraction
- Natural Product Isolation (2) - Purification Techniques, An Overview
- Structure Determination Case Study: Deer Tarsal Gland Pheromone
17 Dienes and MO Theory
- What To Expect In Organic Chemistry 2
- Are these molecules conjugated?
- Conjugation And Resonance In Organic Chemistry
- Bonding And Antibonding Pi Orbitals
- Molecular Orbitals of The Allyl Cation, Allyl Radical, and Allyl Anion
- Pi Molecular Orbitals of Butadiene
- Reactions of Dienes: 1,2 and 1,4 Addition
- Thermodynamic and Kinetic Products
- More On 1,2 and 1,4 Additions To Dienes
- s-cis and s-trans
- The Diels-Alder Reaction
- Cyclic Dienes and Dienophiles in the Diels-Alder Reaction
- Stereochemistry of the Diels-Alder Reaction
- Exo vs Endo Products In The Diels Alder: How To Tell Them Apart
- HOMO and LUMO In the Diels Alder Reaction
- Why Are Endo vs Exo Products Favored in the Diels-Alder Reaction?
- Diels-Alder Reaction: Kinetic and Thermodynamic Control
- The Retro Diels-Alder Reaction
- The Intramolecular Diels Alder Reaction
- Regiochemistry In The Diels-Alder Reaction
- The Cope and Claisen Rearrangements
- Electrocyclic Reactions
- Electrocyclic Ring Opening And Closure (2) - Six (or Eight) Pi Electrons
- Diels Alder Practice Problems
- Molecular Orbital Theory Practice
18 Aromaticity
- Introduction To Aromaticity
- Rules For Aromaticity
- Huckel's Rule: What Does 4n+2 Mean?
- Aromatic, Non-Aromatic, or Antiaromatic? Some Practice Problems
- Antiaromatic Compounds and Antiaromaticity
- The Pi Molecular Orbitals of Benzene
- The Pi Molecular Orbitals of Cyclobutadiene
- Frost Circles
- Aromaticity Practice Quizzes
19 Reactions of Aromatic Molecules
- Electrophilic Aromatic Substitution: Introduction
- Activating and Deactivating Groups In Electrophilic Aromatic Substitution
- Electrophilic Aromatic Substitution - The Mechanism
- Ortho-, Para- and Meta- Directors in Electrophilic Aromatic Substitution
- Understanding Ortho, Para, and Meta Directors
- Why are halogens ortho- para- directors?
- Disubstituted Benzenes: The Strongest Electron-Donor "Wins"
- Electrophilic Aromatic Substitutions (1) - Halogenation of Benzene
- Electrophilic Aromatic Substitutions (2) - Nitration and Sulfonation
- EAS Reactions (3) - Friedel-Crafts Acylation and Friedel-Crafts Alkylation
- Intramolecular Friedel-Crafts Reactions
- Nucleophilic Aromatic Substitution (NAS)
- Nucleophilic Aromatic Substitution (2) - The Benzyne Mechanism
- Reactions on the "Benzylic" Carbon: Bromination And Oxidation
- The Wolff-Kishner, Clemmensen, And Other Carbonyl Reductions
- More Reactions on the Aromatic Sidechain: Reduction of Nitro Groups and the Baeyer Villiger
- Aromatic Synthesis (1) - "Order Of Operations"
- Synthesis of Benzene Derivatives (2) - Polarity Reversal
- Aromatic Synthesis (3) - Sulfonyl Blocking Groups
- Birch Reduction
- Synthesis (7): Reaction Map of Benzene and Related Aromatic Compounds
- Aromatic Reactions and Synthesis Practice
- Electrophilic Aromatic Substitution Practice Problems
20 Aldehydes and Ketones
- What's The Alpha Carbon In Carbonyl Compounds?
- Nucleophilic Addition To Carbonyls
- Aldehydes and Ketones: 14 Reactions With The Same Mechanism
- Sodium Borohydride (NaBH4) Reduction of Aldehydes and Ketones
- Grignard Reagents For Addition To Aldehydes and Ketones
- Wittig Reaction
- Hydrates, Hemiacetals, and Acetals
- Imines - Properties, Formation, Reactions, and Mechanisms
- All About Enamines
- Breaking Down Carbonyl Reaction Mechanisms: Reactions of Anionic Nucleophiles (Part 2)
- Aldehydes Ketones Reaction Practice
21 Carboxylic Acid Derivatives
- Nucleophilic Acyl Substitution (With Negatively Charged Nucleophiles)
- Addition-Elimination Mechanisms With Neutral Nucleophiles (Including Acid Catalysis)
- Basic Hydrolysis of Esters - Saponification
- Transesterification
- Proton Transfer
- Fischer Esterification - Carboxylic Acid to Ester Under Acidic Conditions
- Lithium Aluminum Hydride (LiAlH4) For Reduction of Carboxylic Acid Derivatives
- LiAlH[Ot-Bu]3 For The Reduction of Acid Halides To Aldehydes
- Di-isobutyl Aluminum Hydride (DIBAL) For The Partial Reduction of Esters and Nitriles
- Amide Hydrolysis
- Thionyl Chloride (SOCl2) And Conversion of Carboxylic Acids to Acid Halides
- Diazomethane (CH2N2)
- Carbonyl Chemistry: Learn Six Mechanisms For the Price Of One
- Making Music With Mechanisms (PADPED)
- Carboxylic Acid Derivatives Practice Questions
22 Enols and Enolates
- Keto-Enol Tautomerism
- Enolates - Formation, Stability, and Simple Reactions
- Kinetic Versus Thermodynamic Enolates
- Aldol Addition and Condensation Reactions
- Reactions of Enols - Acid-Catalyzed Aldol, Halogenation, and Mannich Reactions
- Claisen Condensation and Dieckmann Condensation
- Decarboxylation
- The Malonic Ester and Acetoacetic Ester Synthesis
- The Michael Addition Reaction and Conjugate Addition
- The Robinson Annulation
- Haloform Reaction
- The Hell–Volhard–Zelinsky Reaction
- Enols and Enolates Practice Quizzes
23 Amines
- The Amide Functional Group: Properties, Synthesis, and Nomenclature
- Basicity of Amines And pKaH
- 5 Key Basicity Trends of Amines
- The Mesomeric Effect And Aromatic Amines
- Nucleophilicity of Amines
- Alkylation of Amines (Sucks!)
- Reductive Amination
- The Gabriel Synthesis
- Some Reactions of Azides
- The Hofmann Elimination
- The Hofmann and Curtius Rearrangements
- The Cope Elimination
- Protecting Groups for Amines - Carbamates
- The Strecker Synthesis of Amino Acids
- Introduction to Peptide Synthesis
- Reactions of Diazonium Salts: Sandmeyer and Related Reactions
- Amine Practice Questions
24 Carbohydrates
- D and L Notation For Sugars
- Pyranoses and Furanoses: Ring-Chain Tautomerism In Sugars
- What is Mutarotation?
- Reducing Sugars
- The Big Damn Post Of Carbohydrate-Related Chemistry Definitions
- The Haworth Projection
- Converting a Fischer Projection To A Haworth (And Vice Versa)
- Reactions of Sugars: Glycosylation and Protection
- The Ruff Degradation and Kiliani-Fischer Synthesis
- Isoelectric Points of Amino Acids (and How To Calculate Them)
- Carbohydrates Practice
- Amino Acid Quizzes
25 Fun and Miscellaneous
- A Gallery of Some Interesting Molecules From Nature
- Screw Organic Chemistry, I'm Just Going To Write About Cats
- On Cats, Part 1: Conformations and Configurations
- On Cats, Part 2: Cat Line Diagrams
- On Cats, Part 4: Enantiocats
- On Cats, Part 6: Stereocenters
- Organic Chemistry Is Shit
- The Organic Chemistry Behind "The Pill"
- Maybe they should call them, "Formal Wins" ?
- Why Do Organic Chemists Use Kilocalories?
- The Principle of Least Effort
- Organic Chemistry GIFS - Resonance Forms
- Reproducibility In Organic Chemistry
- What Holds The Nucleus Together?
- How Reactions Are Like Music
- Organic Chemistry and the New MCAT
26 Organic Chemistry Tips and Tricks
- Common Mistakes: Formal Charges Can Mislead
- Partial Charges Give Clues About Electron Flow
- Draw The Ugly Version First
- Organic Chemistry Study Tips: Learn the Trends
- The 8 Types of Arrows In Organic Chemistry, Explained
- Top 10 Skills To Master Before An Organic Chemistry 2 Final
- Common Mistakes with Carbonyls: Carboxylic Acids... Are Acids!
- Planning Organic Synthesis With "Reaction Maps"
- Alkene Addition Pattern #1: The "Carbocation Pathway"
- Alkene Addition Pattern #2: The "Three-Membered Ring" Pathway
- Alkene Addition Pattern #3: The "Concerted" Pathway
- Number Your Carbons!
- The 4 Major Classes of Reactions in Org 1
- How (and why) electrons flow
- Grossman's Rule
- Three Exam Tips
- A 3-Step Method For Thinking Through Synthesis Problems
- Putting It Together
- Putting Diels-Alder Products in Perspective
- The Ups and Downs of Cyclohexanes
- The Most Annoying Exceptions in Org 1 (Part 1)
- The Most Annoying Exceptions in Org 1 (Part 2)
- The Marriage May Be Bad, But the Divorce Still Costs Money
- 9 Nomenclature Conventions To Know
- Nucleophile attacks Electrophile
27 Case Studies of Successful O-Chem Students
- Success Stories: How Corina Got The The "Hard" Professor - And Got An A+ Anyway
- How Helena Aced Organic Chemistry
- From a "Drop" To B+ in Org 2 – How A Hard Working Student Turned It Around
- How Serge Aced Organic Chemistry
- Success Stories: How Zach Aced Organic Chemistry 1
- Success Stories: How Kari Went From C– to B+
- How Esther Bounced Back From a "C" To Get A's In Organic Chemistry 1 And 2
- How Tyrell Got The Highest Grade In Her Organic Chemistry Course
- This Is Why Students Use Flashcards
- Success Stories: How Stu Aced Organic Chemistry
- How John Pulled Up His Organic Chemistry Exam Grades
- Success Stories: How Nathan Aced Organic Chemistry (Without It Taking Over His Life)
- How Chris Aced Org 1 and Org 2
- Interview: How Jay Got an A+ In Organic Chemistry
- How to Do Well in Organic Chemistry: One Student's Advice
- "America's Top TA" Shares His Secrets For Teaching O-Chem
- "Organic Chemistry Is Like..." - A Few Metaphors
- How To Do Well In Organic Chemistry: Advice From A Tutor
- Guest post: "I went from being afraid of tests to actually looking forward to them".
For the methyl cyclohexanol example, shouldn’t the dash be inverted into wedge when ether is formed?
I had a similar question to the one above–bulky bases are supposed to be an example of a poor nucleophile but a really strong base, so cause E2 reactions, with Hoffmann elimination when applicable for steric reasons. But the textbook and this site also say that using a tert-butoxide ion is a better bet for making an ether by SN2 reaction than say, a tertiary alkyl halide in which case only elimination product comes.
But then, I guess you could make an ether by SN1 reaction on a tertiary alkyl halide? As you said in a response to another comment. The textbook says the reaction of CH3ONa with (CH3)3C-Br gives exclusively 2-methylpropene. Guess that’s an experimental result.
Source:
https://flic.kr/p/2ogyr3S
https://flic.kr/p/2ogxHyZ
NCERT chemistry class 12
Will reaction of sodium tert-butoxide and chloroethane give ethene (due to E2) or t-butyl ethyl ether (due to SN2) as major product?
Mechanism for Williamson synthesis
You are right that it isn’t in there. Need to fix that. It’s just an SN2.
Thanks for sharing with such useful details.
If the product is water insoluble, you can get rid of excess base simply by pouring the reaction in water; however, my product is highly water soluble, do you have any suggestions how to separate it from the excess base? (I use K2CO3)
Is your product soluble in any organic solvents at all? One way to do it would be to quench the base with saturated NH4Cl solution, and then add equal volumes of brine and n-BuOH. Perform 3 extractions with n-BuOH and your organic molecule should persist in that layer while all the salts will be in the aqueous layer.
Is it possible to use NaOH instead of NaH as a base in the formation reaction of alcohol to Alkoxide?
Sure! There will be an equilibrium between alkoxide and alcohol but will still get the job done. It’s best when the solvent is the conjugate acid of the alkoxide (e.g. EtO- / EtOH).
hello and thank you very much for your notes, I found them really helpful.
I have a question: in my project I had to form a benzylic ether to protect a phenol. I did it using NaI, K2CO3 and BnBr in DMF at 80oC as literature suggested. Is this a Williamson synthesis and does the ether form through an Sn2? If yes then why NaI and K2CO3 and not a stronger base?
The NaI makes benzyl iodide from benzyl bromide. Benzyl iodide is too unstable to isolate.
The pKa of phenol is 10. The pKa of carbonic acid is about 6. The pKa difference is 4. A good rule of thumb is that a pKa difference of 8 or less will be sufficient to get your conjugate base to participate. So although the acid-base reaction will like far on the carbonate side, there will be enough phenoxide to react with your benzyl iodide.
Can we please tell me if SN1 is possible in Williamson ether synthesis?..Because our Teacher today said it can… And i have learned otherwise 😕..Iam a 12th standard student
*ether formation* is possible via SN1. For example, take a tertiary alkyl halide like t-butyl bromide and dissolve in methanol; you’ll get a new ether, t butyl methyl ether.
However that’s not technically a Williamson; a Williamson involves deprotonating an alcohol to give an alkoxide, and then having that react with an alkyl halide to give a new ether. This proceeds through an SN2 reaction.
Ultimately the name “Williamson” isn’t very important; what’s more important is to realize that ethers can form both through SN1 and SN2 reactions.
Hii, I have a dought. Is that possible of good yield with a primary alcohol and a secondary halide having steric hindered groups ?. I tried with a strong base in polar solvent but yield was very less 6-7% with unreacted SM.
The SN2 with secondary alkyl halides, particularly hindered secondary alkyl halides, is quite poor. Why don’t you switch it around and use a secondary alkoxide with a primary alkyl halide? That would work much better.
Hi James,
According to you, the following sequence :
1) NaH
2) BnBr
is a SN1 or SN2 mechanism ?
Thanks for your answer
Phil
I don’t see the molecules you’re working on, but I assume that NaH is to deprotonate an alcohol, and the BnBr reacts with the resulting alkoxide.
Hi, I learned a lot in this page. However, could you give some more examples about the conjugate acid of the alkoxide while choosing solvent.
Hello! I have a question: why heptanol can not be deprtonated by NaOH?
It can, it’s just that it will be highly reversible. you might have some solubility issues as well as heptanol is on the greasier side of things. Try a phase transfer catalyst. Tetraammonium hydroxide
Outstanding Presentations.
Found your web pages while helping my Daughter find good sources of info for her Organic course.
Concise but not Terse…
Pros, Cons, Comparisons, Rationale for choosing Reactant-Substrates with Reagents to yield preferred products along with related ” Be Aware Of This” notations are on point.
Your presentations perfectly full fill my Golidlocks criteria for selecting a Professors and Teachers.
Not To Little
Not Too Much,
PERFECT Presentation
Tom Jones, D.D.S.
Thank you
Can we use K2CO3 as a base to make the alkoxide ?
Not a great base to use because it’s quite weak. Equilibrium greatly favours the alcohol, not the alkoxide.
It depends also on the irreversibility, the extrusion of CO2 may help; maybe you need then the alkyliodide ….
Excellent stuff! World needs authors like you in chemistry! This is how an information should be conveyed. Short & covered all essential points..
Using DMSO(aprotic solvent) will favour E2 and not Sn2.Instead of Aprotic,protic solvent should be used.
This is not correct. Aprotic solvents favor SN2 over E2, and DMSO is a useful solvent for an SN2 reaction. Think of it this way. A polar PROTIC solvent would hydrogen bond to the nucleophile and hinder its backside attack.
“… after the base does its deprotonation, its conjugate base is still swimming around in solution…”
“it is non-nucloephilic”
“Easily avoided if we we just…”
Please make the appropriate corrections.
But a really great and useful post, actually (well, as usual).
Glad the mistakes are minor this time. Thank you, as always.